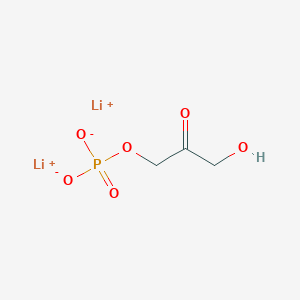
Dihydroxyacetone phosphate dilithium salt
Overview
Description
Dihydroxyacetone phosphate dilithium salt is a chemical compound with the empirical formula C₃H₅Li₂O₆P. It is a metabolic intermediate involved in various biochemical pathways, including glycolysis, gluconeogenesis, glycerol metabolism, phosphatidic acid synthesis, fat metabolism, and the Calvin cycle .
Mechanism of Action
Target of Action
Dihydroxyacetone phosphate (DHAP) primarily targets enzymes such as fructose-bisphosphate aldolase (ALDOA) and triosephosphate isomerase . These enzymes play a crucial role in various metabolic pathways, including glycolysis and gluconeogenesis .
Mode of Action
DHAP interacts with its target enzymes by serving as a substrate. It is used in studies involving the metabolism of three-carbon sugar pools and their roles in physiological and physical processes . The interaction of DHAP with these enzymes leads to various biochemical reactions that are essential for energy production and other metabolic processes .
Biochemical Pathways
DHAP is a metabolic intermediate involved in many pathways, including glycolysis, gluconeogenesis, glycerol metabolism, phosphatidic acid synthesis, fat metabolism, and the Calvin cycle . In glycolysis, for instance, DHAP is converted to glyceraldehyde 3-phosphate, which then undergoes further reactions to produce ATP, the cell’s main energy currency .
Result of Action
The action of DHAP at the molecular and cellular levels results in the production of energy and the regulation of various metabolic processes. By serving as a substrate for key enzymes, DHAP facilitates the breakdown of glucose and the synthesis of other important molecules, contributing to the overall metabolic functioning of the cell .
Biochemical Analysis
Biochemical Properties
Dihydroxyacetone phosphate dilithium salt plays a crucial role in biochemical reactions. It interacts with various enzymes, proteins, and other biomolecules. For instance, it may be used as a substrate to help identify, differentiate, and characterize fructose-bisphosphate aldolase (ALDOA) and triosephosphate isomerase . The nature of these interactions is essential for the metabolism of three-carbon sugar pools and their roles in physiological and physical processes .
Cellular Effects
This compound has significant effects on various types of cells and cellular processes. It influences cell function, including any impact on cell signaling pathways, gene expression, and cellular metabolism . For example, it may be used to study cellular glycation stress .
Molecular Mechanism
The molecular mechanism of action of this compound involves its effects at the molecular level, including any binding interactions with biomolecules, enzyme inhibition or activation, and changes in gene expression . It is rapidly and reversibly isomerised to glyceraldehyde 3-phosphate .
Temporal Effects in Laboratory Settings
The effects of this compound change over time in laboratory settings. Information on the product’s stability, degradation, and any long-term effects on cellular function observed in in vitro or in vivo studies is crucial for understanding its biochemical properties .
Dosage Effects in Animal Models
The effects of this compound vary with different dosages in animal models. This includes any threshold effects observed in these studies, as well as any toxic or adverse effects at high doses .
Metabolic Pathways
This compound is involved in several metabolic pathways. It interacts with various enzymes or cofactors, and this interaction can affect metabolic flux or metabolite levels . For instance, it is involved in the Calvin cycle in plants and glycolysis .
Transport and Distribution
This compound is transported and distributed within cells and tissues. It could interact with transporters or binding proteins, affecting its localization or accumulation .
Subcellular Localization
The subcellular localization of this compound and any effects on its activity or function are crucial for understanding its biochemical properties. This could include any targeting signals or post-translational modifications that direct it to specific compartments or organelles .
Preparation Methods
Synthetic Routes and Reaction Conditions
Dihydroxyacetone phosphate dilithium salt can be synthesized through both chemical and enzymatic routes. Chemical synthetic routes often focus on producing a storable precursor that can be easily converted to dihydroxyacetone phosphate immediately before its use . One such method involves the Lewis acid-mediated regioselective epoxide ring opening with dibenzyl phosphate, followed by catalytic oxidation of the secondary alcohol by tetrapropylammonium perruthenate/N-methylmorpholine N-oxide, leading to dibenzyl-3-benzylhydroxyacetone phosphate. This stable stock material is then quantitatively hydrogenolyzed into dihydroxyacetone phosphate .
Enzymatic routes typically involve integrating the formation of dihydroxyacetone phosphate with upstream or downstream catalytic steps, leading to multi-enzyme arrangements with up to seven enzymes operating simultaneously . These routes often start from cheap unphosphorylated precursors such as dihydroxyacetone or glycerol, which are then converted to dihydroxyacetone phosphate through a series of enzymatic reactions .
Industrial Production Methods
Industrial production of this compound often relies on enzymatic methods due to their mild reaction conditions and suitability for multifunctional complex molecules . These methods are scalable and can be integrated into existing biochemical production processes.
Chemical Reactions Analysis
Types of Reactions
Dihydroxyacetone phosphate dilithium salt undergoes various types of chemical reactions, including:
Oxidation: Catalytic oxidation of secondary alcohols.
Reduction: Reduction of ketone groups.
Substitution: Nucleophilic substitution reactions involving phosphate groups.
Common Reagents and Conditions
Common reagents used in these reactions include:
Oxidizing agents: Tetrapropylammonium perruthenate/N-methylmorpholine N-oxide.
Reducing agents: Sodium borohydride.
Nucleophiles: Dibenzyl phosphate.
Major Products
Major products formed from these reactions include various phosphorylated intermediates and derivatives of dihydroxyacetone phosphate .
Scientific Research Applications
Dihydroxyacetone phosphate dilithium salt is widely used in scientific research due to its role as a metabolic intermediate. Its applications include:
Comparison with Similar Compounds
Similar Compounds
Dihydroxyacetone phosphate lithium salt: Similar in structure and function but differs in the cation present.
Dihydroxyacetone phosphate hemimagnesium salt hydrate: Another variant with magnesium as the cation.
Uniqueness
Dihydroxyacetone phosphate dilithium salt is unique due to its specific cation composition, which can influence its solubility and reactivity in biochemical reactions. This makes it particularly suitable for certain enzymatic processes and industrial applications .
Biological Activity
Dihydroxyacetone phosphate dilithium salt (DHAP) is a critical metabolic intermediate involved in various biochemical pathways, including glycolysis and gluconeogenesis. This article provides an in-depth exploration of its biological activity, including case studies, research findings, and a summary of its applications in enzymatic assays and metabolic studies.
Dihydroxyacetone phosphate (DHAP) is a zwitterionic compound characterized by the presence of both positive and negative charges. The dilithium salt form enhances its solubility and stability in biological assays. The molecular formula for DHAP is with a molecular weight of approximately 181.92 g/mol. Its structural representation includes:
- Functional Groups : Hydroxyl groups (-OH), phosphate group (-PO₄), and a ketone group (C=O).
The compound is soluble in water, forming a clear solution upon dissolution.
Role in Metabolism
DHAP plays a pivotal role in carbohydrate metabolism:
- Glycolysis : DHAP is produced from fructose 1,6-bisphosphate through the action of aldolase enzymes. It can be converted into glyceraldehyde 3-phosphate (G3P), which continues through the glycolytic pathway to produce energy.
- Gluconeogenesis : In the synthesis of glucose from non-carbohydrate precursors, DHAP serves as an intermediate, highlighting its importance in maintaining glucose homeostasis.
Enzymatic Assays and Applications
DHAP is widely utilized as a substrate in various enzymatic assays, particularly those involving triosephosphate isomerase (TPI). Its role as a substrate allows researchers to study enzyme kinetics and mechanisms associated with carbohydrate metabolism.
Table 1: Summary of Enzymatic Applications
Enzyme | Function | Substrate |
---|---|---|
Triosephosphate Isomerase (TPI) | Converts DHAP to G3P | Dihydroxyacetone phosphate |
Aldolase | Catalyzes the condensation of DHAP with G3P | Dihydroxyacetone phosphate |
Glyceraldehyde 3-Phosphate Dehydrogenase (GAPDH) | Involved in glycolysis, utilizing G3P | Glyceraldehyde 3-phosphate |
Case Studies
- In Vitro Glycation Studies : A study investigated the glycation of human serum albumin (HSA) by DHAP, revealing that DHAP can participate in the formation of advanced glycation end-products (AGEs). This has implications for understanding diabetic complications where AGEs play a significant role .
- Enzyme Inhibition Research : Research has highlighted the potential of DHAP as a competitive substrate for class II fructose 1,6-bisphosphate aldolases (FBAs). This enzyme's inhibition by compounds mimicking DHAP suggests therapeutic avenues for targeting bacterial pathogens .
- Metabolic Profiling : A study involving vitamin D deficiency examined the metabolic profiles associated with obesity, utilizing DHAP as part of the analysis to differentiate between obese and non-obese subjects .
Research Findings
Recent studies have shown that alterations in DHAP levels can influence various metabolic pathways:
- Reactive Carbonyl Species : Research indicates that reactive carbonyls generated during metabolic processes can interact with DHAP, potentially leading to cellular damage .
- Antibacterial Targets : The structural dynamics of FBAs interacting with DHAP have been studied to identify potential antibacterial targets against pathogens like Mycobacterium tuberculosis .
Properties
IUPAC Name |
dilithium;(3-hydroxy-2-oxopropyl) phosphate | |
---|---|---|
Source | PubChem | |
URL | https://pubchem.ncbi.nlm.nih.gov | |
Description | Data deposited in or computed by PubChem | |
InChI |
InChI=1S/C3H7O6P.2Li/c4-1-3(5)2-9-10(6,7)8;;/h4H,1-2H2,(H2,6,7,8);;/q;2*+1/p-2 | |
Source | PubChem | |
URL | https://pubchem.ncbi.nlm.nih.gov | |
Description | Data deposited in or computed by PubChem | |
InChI Key |
QWIKESRFRWLYIA-UHFFFAOYSA-L | |
Source | PubChem | |
URL | https://pubchem.ncbi.nlm.nih.gov | |
Description | Data deposited in or computed by PubChem | |
Canonical SMILES |
[Li+].[Li+].C(C(=O)COP(=O)([O-])[O-])O | |
Source | PubChem | |
URL | https://pubchem.ncbi.nlm.nih.gov | |
Description | Data deposited in or computed by PubChem | |
Molecular Formula |
C3H5Li2O6P | |
Source | PubChem | |
URL | https://pubchem.ncbi.nlm.nih.gov | |
Description | Data deposited in or computed by PubChem | |
DSSTOX Substance ID |
DTXSID10585136 | |
Record name | Dilithium 3-hydroxy-2-oxopropyl phosphate | |
Source | EPA DSSTox | |
URL | https://comptox.epa.gov/dashboard/DTXSID10585136 | |
Description | DSSTox provides a high quality public chemistry resource for supporting improved predictive toxicology. | |
Molecular Weight |
182.0 g/mol | |
Source | PubChem | |
URL | https://pubchem.ncbi.nlm.nih.gov | |
Description | Data deposited in or computed by PubChem | |
CAS No. |
102783-56-2 | |
Record name | Dilithium 3-hydroxy-2-oxopropyl phosphate | |
Source | EPA DSSTox | |
URL | https://comptox.epa.gov/dashboard/DTXSID10585136 | |
Description | DSSTox provides a high quality public chemistry resource for supporting improved predictive toxicology. | |
Retrosynthesis Analysis
AI-Powered Synthesis Planning: Our tool employs the Template_relevance Pistachio, Template_relevance Bkms_metabolic, Template_relevance Pistachio_ringbreaker, Template_relevance Reaxys, Template_relevance Reaxys_biocatalysis model, leveraging a vast database of chemical reactions to predict feasible synthetic routes.
One-Step Synthesis Focus: Specifically designed for one-step synthesis, it provides concise and direct routes for your target compounds, streamlining the synthesis process.
Accurate Predictions: Utilizing the extensive PISTACHIO, BKMS_METABOLIC, PISTACHIO_RINGBREAKER, REAXYS, REAXYS_BIOCATALYSIS database, our tool offers high-accuracy predictions, reflecting the latest in chemical research and data.
Strategy Settings
Precursor scoring | Relevance Heuristic |
---|---|
Min. plausibility | 0.01 |
Model | Template_relevance |
Template Set | Pistachio/Bkms_metabolic/Pistachio_ringbreaker/Reaxys/Reaxys_biocatalysis |
Top-N result to add to graph | 6 |
Feasible Synthetic Routes
Disclaimer and Information on In-Vitro Research Products
Please be aware that all articles and product information presented on BenchChem are intended solely for informational purposes. The products available for purchase on BenchChem are specifically designed for in-vitro studies, which are conducted outside of living organisms. In-vitro studies, derived from the Latin term "in glass," involve experiments performed in controlled laboratory settings using cells or tissues. It is important to note that these products are not categorized as medicines or drugs, and they have not received approval from the FDA for the prevention, treatment, or cure of any medical condition, ailment, or disease. We must emphasize that any form of bodily introduction of these products into humans or animals is strictly prohibited by law. It is essential to adhere to these guidelines to ensure compliance with legal and ethical standards in research and experimentation.