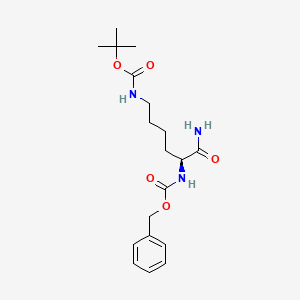
Z-Lys(boc)-NH2
Overview
Description
Z-Lys(Boc)-NH2 (N-α-carbobenzoxy-N-ε-(tert-butoxycarbonyl)-L-lysine amide) is a dual-protected lysine derivative widely used in peptide synthesis and polymer chemistry. Its structure features two orthogonal protecting groups:
- Z (carbobenzoxy): Removed via catalytic hydrogenation or acidolysis.
- Boc (tert-butoxycarbonyl): Cleaved under acidic conditions (e.g., trifluoroacetic acid, TFA) .
With a molecular weight of 379.46 g/mol , this compound is valued for its stability during stepwise syntheses, enabling selective deprotection. It is synthesized through coupling reactions using activating agents like DCC (dicyclohexylcarbodiimide) and HOSu (N-hydroxysuccinimide) in solvents such as DMF or THF . The amide (-NH2) terminus allows direct incorporation into peptide chains without additional activation, distinguishing it from carboxylic acid analogs like Z-Lys(Boc)-OH.
Scientific Research Applications
Peptide Synthesis
1.1 Conventional Peptide Coupling
Z-Lys(Boc)-NH2 serves as a key building block in the synthesis of peptides through conventional coupling methods. The Boc group provides protection for the amino group during synthesis, allowing for selective reactions. For instance, this compound can be coupled with other amino acids to create complex peptide sequences. Studies have demonstrated its effectiveness in synthesizing ligands for various biological applications, including those targeting specific receptors or enzymes .
1.2 Side-Chain Modifications
The versatility of this compound extends to the modification of peptide side chains. Researchers have successfully utilized this compound to create side-chain modified polypeptides that exhibit enhanced solubility and biological activity. For example, the incorporation of this compound into polymeric structures has led to the development of water-soluble peptides with α-helical conformations, which are crucial for biological function .
Drug Development
2.1 Opioid Pharmacology
this compound has been employed in the design of opioid peptides. For instance, modifications using this compound have resulted in new μ-opioid antagonists that show promise as pharmacological tools in opioid research. These compounds have been tested for their binding affinities and selectivity, revealing significant potential for therapeutic applications .
2.2 Targeted Drug Delivery
In drug delivery systems, this compound is utilized to create targeted ligands that enhance the specificity of drug action. By linking this compound with other functional groups, researchers have developed ligands that can selectively bind to target cells or tissues, improving therapeutic outcomes while minimizing side effects .
Bioconjugation Techniques
3.1 Formation of Bioconjugates
this compound is instrumental in bioconjugation techniques where it acts as a linker between biomolecules. Its reactive amine group allows for the attachment of various entities such as drugs, fluorophores, or other peptides, facilitating the creation of multifunctional biomolecules that can be used in diagnostics and therapeutics .
3.2 Enzyme Inhibition Studies
Research has shown that derivatives of this compound can be used to study enzyme inhibition mechanisms. By incorporating this compound into inhibitor designs, scientists can explore interactions at the molecular level, leading to insights into enzyme functionality and potential therapeutic strategies .
Data Tables and Case Studies
Application Area | Description | Key Findings |
---|---|---|
Peptide Synthesis | Building block for peptide synthesis | Effective coupling with various amino acids |
Opioid Pharmacology | Development of μ-opioid antagonists | Promising binding affinities observed |
Targeted Drug Delivery | Creation of selective ligands | Improved specificity and reduced side effects |
Bioconjugation Techniques | Linker for biomolecule attachment | Enhanced functionality in diagnostics |
Enzyme Inhibition Studies | Study of enzyme interactions | Insights into molecular interactions |
Mechanism of Action
The mechanism by which Z-Lys(boc)-NH2 exerts its effects depends on its incorporation into peptides and proteins. The lysine residue can interact with various molecular targets, influencing cellular processes such as gene transcription, cell differentiation, and apoptosis.
Comparison with Similar Compounds
Structural and Functional Differences
Z-Lys(Boc)-OH
- Structure : Contains a carboxylic acid (-OH) instead of an amide.
- Applications : Used in dendrimer synthesis (e.g., coupling to core compounds) and polymerizations (e.g., polylysine via N-carboxyanhydrides) .
- Reactivity : Requires activation (e.g., Pfp esters) for peptide bond formation, unlike Z-Lys(Boc)-NH2 .
Boc-Lys(Z)-OH
- Isotope Variants : Used in isotopic labeling (e.g., α-15N for NMR studies) .
- Deprotection: Sequential removal of Boc (TFA) and Z (hydrogenolysis) mirrors this compound but lacks the amide functionality .
Z-Lys(NH2)AMC and Z-Lys(HMG)AMC
- Structure: Feature AMC (7-amino-4-methylcoumarin) fluorophores.
- Synthesis : Z-Lys(NH2)AMC achieves 93% yield, while Z-Lys(HMG)AMC yields 32%, indicating steric challenges with bulkier HMG groups .
- Applications : Fluorescent probes for enzymatic assays due to AMC's detectable cleavage .
Z-Pro-Lys(Boc)-Pro-Val-NH2
- Structure : Tetrapeptide incorporating Z-Lys(Boc) as a central residue.
- Molecular Weight : 672.822 g/mol, highlighting its role in therapeutic peptide design .
- Synthesis : Demonstrates this compound’s utility in complex peptide architectures .
Comparative Data Table
Reactivity and Stability
- Orthogonal Protection : this compound’s dual protection enables sequential deprotection, critical for multi-step syntheses. In contrast, Z-Lys(Fmoc)-OH () requires base for Fmoc removal, limiting compatibility with acid-labile substrates.
- Side Reactions : TFA treatment of Z-protected compounds risks N-benzylation (e.g., 3.13% in N'-Z-Lys), necessitating careful optimization .
- Polymerization Suitability : this compound’s amide group precludes N-carboxyanhydride (NCA) formation, unlike Z-Lys(Boc)-OH, which is polymerized into polylysine .
Biological Activity
Z-Lys(boc)-NH2, also known as Z-lysine (Boc)-amide, is a derivative of lysine that has garnered attention in various biological studies due to its potential therapeutic applications. This article explores the biological activity of this compound, focusing on its antimicrobial, anticancer, and cytotoxic properties, supported by data tables and relevant case studies.
Chemical Structure and Properties
This compound is characterized by the presence of a benzyloxycarbonyl (Z) protecting group on the amino group of lysine, which enhances its stability and solubility in biological systems. The molecular formula is C19H29N3O5, and it has a molecular weight of 389.45 g/mol .
Antimicrobial Activity
Recent studies indicate that this compound exhibits significant antimicrobial activity against various bacterial strains. For instance, research involving synthetic peptides containing lysine derivatives showed promising results against Escherichia coli and other Gram-negative bacteria.
Table 1: Antimicrobial Activity of this compound Derivatives
Compound | Bacterial Strain | Minimum Inhibitory Concentration (MIC) |
---|---|---|
This compound | E. coli K12 | 20 µM |
(KLAKLAK)2-NH2 | E. coli K12 | 10 µM |
KLβAKLβAK-NH2 | Staphylococcus aureus | 15 µM |
The introduction of β-alanine in the structure of related compounds has been shown to enhance their antimicrobial efficacy significantly .
Anticancer Properties
This compound and its analogs have been investigated for their anticancer properties. Studies demonstrate that certain derivatives exhibit selective cytotoxicity towards cancer cell lines such as MCF-7 (breast cancer) and HeLa (cervical cancer).
Case Study: Cytotoxicity Evaluation
In a study assessing the cytotoxic effects of this compound derivatives on MCF-7 cells, researchers reported:
- Compound Tested : this compound
- Cell Line : MCF-7
- IC50 Value : 25 µM after 48 hours of treatment.
This suggests that this compound can inhibit cell proliferation effectively at relatively low concentrations .
The biological activity of this compound can be attributed to its ability to interact with cellular membranes and proteins. The positively charged amine group may facilitate binding to negatively charged bacterial membranes or cancer cell surfaces, leading to disruption and subsequent cell death.
Table 2: Mechanisms Involved in Biological Activity
Mechanism | Description |
---|---|
Membrane Disruption | Alters membrane integrity leading to cell lysis. |
Protein Interaction | Binds to specific proteins involved in cell signaling pathways. |
Induction of Apoptosis | Triggers programmed cell death in cancer cells. |
Chemical Reactions Analysis
Protection Steps
-
ε-Amino Boc Protection :
The ε-amino group of lysine is protected using di-tert-butyl dicarbonate (Boc₂O) under basic conditions (e.g., aqueous NaHCO₃ or DIEA) . -
α-Amino Z Protection :
The α-amino group is protected with benzyloxycarbonyl (Z) chloride in the presence of a base (e.g., NaOH) .
Deprotection Conditions
Key Findings :
-
Boc removal with TFA requires scavengers (e.g., thioanisole) to suppress N-benzylation via carbonium ion intermediates .
-
Z-group stability in acidic conditions allows sequential deprotection strategies .
Stability and Intramolecular Interactions
The Boc and Z groups influence conformational stability through intramolecular hydrogen bonding:
FTIR and NMR Analysis
Compound | NH Stretching (cm⁻¹) | CO Stretching (cm⁻¹) | Hydrogen Bonding Interactions |
---|---|---|---|
Z-Lys(Boc)-NH₂ | 3449 (free NH) | 1652 (Boc CO) | NH (Lys2) → CO (Boc) β-turn |
3375 (bound NH) | 1734 (Z CO) | NεH (Lys) → CO (azaPhe) |
Key Findings :
-
Boc’s carbonyl forms a pseudocyclic 10-atom hydrogen bond with NH (Lys2), stabilizing β-turn conformations .
-
Z-group carbonyls remain unbound in dilute solutions, enhancing reactivity for subsequent couplings .
N-Benzylation Under Acidic Conditions
TFA-mediated Boc removal can inadvertently benzylate the ε-amino group if scavengers are omitted:
Reagent | N-Benzylation Yield | Mitigation Strategy |
---|---|---|
TFA (neat) | ≤3.26% | Add thioanisole (2.5%) |
TFA/CH₂Cl₂ (1:1) | ≤0.88% | Use 2,4-Cl₂-Z groups |
Key Insight :
Comparative Stability of Protecting Groups
Parameter | Z Group | Boc Group |
---|---|---|
Acid Stability | Moderate (HBr/AcOH) | High (TFA-labile) |
Hydrogen Bonding | Weak | Strong (β-turn) |
Side Chain Reactivity | Low | High (NεH free post-deprotection) |
Innovative Deprotection Strategies
Q & A
Q. What is the functional role of the Z and Boc protecting groups in Z-Lys(Boc)-NH₂ during peptide synthesis?
Basic Question
The Z (benzyloxycarbonyl) and Boc (tert-butoxycarbonyl) groups protect the α-amino and ε-amino groups of lysine, respectively, during solid-phase or solution-phase peptide synthesis. This dual protection prevents unintended side reactions, enabling sequential coupling of amino acids. The Boc group is acid-labile (removed via trifluoroacetic acid), while the Z group is typically cleaved by catalytic hydrogenation. This orthogonal protection allows selective deprotection, critical for synthesizing complex peptides with controlled lysine incorporation .
Q. How do solvent choice and reaction conditions influence the stability and reactivity of Z-Lys(Boc)-NH₂?
Basic Question
Z-Lys(Boc)-NH₂ exhibits variable solubility and reactivity depending on solvent polarity. Polar aprotic solvents like DMF or dichloromethane enhance solubility and facilitate coupling reactions, while nonpolar solvents (e.g., hexanes) are used in solid-state polymerizations to achieve high molecular weights. Reaction temperatures (e.g., 0–25°C) and pH are critical: acidic conditions risk premature Boc deprotection, while basic conditions may hydrolyze active esters like N-hydroxysuccinimide (NHS) .
Q. What methodological challenges arise in synthesizing Z-Lys(Boc)-NH₂, and how can they be addressed?
Advanced Question
Historically, low yields (<50%) stemmed from incomplete coupling or side reactions during ε-amino protection. Improved strategies include:
- Activated esters : Using NHS or pentafluorophenyl esters with dicyclohexylcarbodiimide (DCC) as a condensing agent, achieving 90–95% coupling efficiency .
- Hazard mitigation : Replacing toxic reagents (e.g., phosgene for N-carboxyanhydrides) with safer alternatives like triphosgene .
- Solid-state ROP : For polymerization, hexylamine-initiated solid-state ring-opening polymerization (ROP) of Z-Lys NTA achieves quantitative conversion in 45 hours, avoiding solubility issues .
Q. How can researchers validate the purity and structural integrity of Z-Lys(Boc)-NH₂ post-synthesis?
Advanced Question
- Chromatography : Reverse-phase HPLC (RP-HPLC) with UV detection identifies impurities, while SEC-MALS-DRI determines polymer molecular weights (8.5–50.0 kDa) .
- Spectroscopy : ¹H/¹³C NMR confirms deprotection efficiency and backbone integrity. For example, Boc group removal via TFA results in characteristic tert-butyl signal loss at δ 1.4 ppm .
- Mass spectrometry : High-resolution MS (HRMS) verifies exact masses, critical for detecting side products like truncated peptides or overprotection .
Q. What are the implications of Z-Lys(Boc)-NH₂ in biochemical engineering beyond peptide synthesis?
Advanced Question
Z-Lys(Boc)-NH₂ enables site-specific incorporation of unnatural amino acids in synthetic biology. For example, engineered E. coli strains with pyrrolysyl-tRNA synthetase incorporate Z-Lys at sense codons, producing proteins with tailored functionalities (e.g., bio-containment systems). The Boc group ensures metabolic stability until deprotection, which triggers controlled cellular toxicity—a safeguard against unintended release of genetically modified organisms .
Q. How can researchers optimize orthogonal deprotection strategies for Z-Lys(Boc)-NH₂ in multi-step syntheses?
Advanced Question
- Sequential deprotection : Catalytic hydrogenation (H₂/Pd-C) removes the Z group first, followed by Boc cleavage with TFA. Methanesulfonic acid (MeSO₃H) is used in situ to stabilize intermediates during hydrogenolysis .
- Solvent compatibility : Avoid protic solvents (e.g., MeOH) during hydrogenation to prevent catalyst poisoning. Anhydrous CH₂Cl₂ or DMF is preferred for acidolytic Boc removal .
- Kinetic monitoring : Real-time FTIR or inline HPLC tracks deprotection progress, minimizing overexposure to harsh conditions that degrade the peptide backbone .
Q. What role does Z-Lys(Boc)-NH₂ play in polymer chemistry, and how are its properties tailored?
Advanced Question
Z-Lys(Boc)-NH₂ serves as a monomer for polylysine derivatives via ROP. Key modifications include:
- Side-chain engineering : The Boc group enhances solubility in organic solvents, enabling polymerization in hexanes. Post-polymerization deprotection yields cationic polylysine for drug delivery .
- Molecular weight control : Initiators like hexylamine and [M]₀/[I]₀ ratios <100:1 produce narrow dispersity (Đ = 1.1–1.3) polymers. SEC-MALS-DRI correlates experimental Mn with theoretical values .
Q. What experimental design considerations are critical when incorporating Z-Lys(Boc)-NH₂ into enzyme-sensitive peptides?
Advanced Question
- Protease resistance : The Boc group shields lysine from trypsin-like proteases, enabling selective cleavage at unprotected residues.
- pH-dependent release : In drug delivery, Boc deprotection in acidic environments (e.g., tumor microenvironments) triggers payload release. Validate using fluorescence quenching assays or MALDI-TOF .
- Compatibility with bioconjugation : NHS esters of Z-Lys(Boc)-NH₂ react selectively with primary amines (e.g., hexamethylenediamine), enabling controlled crosslinking in hydrogels .
Properties
IUPAC Name |
benzyl N-[(2S)-1-amino-6-[(2-methylpropan-2-yl)oxycarbonylamino]-1-oxohexan-2-yl]carbamate | |
---|---|---|
Details | Computed by Lexichem TK 2.7.0 (PubChem release 2021.05.07) | |
Source | PubChem | |
URL | https://pubchem.ncbi.nlm.nih.gov | |
Description | Data deposited in or computed by PubChem | |
InChI |
InChI=1S/C19H29N3O5/c1-19(2,3)27-17(24)21-12-8-7-11-15(16(20)23)22-18(25)26-13-14-9-5-4-6-10-14/h4-6,9-10,15H,7-8,11-13H2,1-3H3,(H2,20,23)(H,21,24)(H,22,25)/t15-/m0/s1 | |
Details | Computed by InChI 1.0.6 (PubChem release 2021.05.07) | |
Source | PubChem | |
URL | https://pubchem.ncbi.nlm.nih.gov | |
Description | Data deposited in or computed by PubChem | |
InChI Key |
NRIVYVKAKANCDC-HNNXBMFYSA-N | |
Details | Computed by InChI 1.0.6 (PubChem release 2021.05.07) | |
Source | PubChem | |
URL | https://pubchem.ncbi.nlm.nih.gov | |
Description | Data deposited in or computed by PubChem | |
Canonical SMILES |
CC(C)(C)OC(=O)NCCCCC(C(=O)N)NC(=O)OCC1=CC=CC=C1 | |
Details | Computed by OEChem 2.3.0 (PubChem release 2021.05.07) | |
Source | PubChem | |
URL | https://pubchem.ncbi.nlm.nih.gov | |
Description | Data deposited in or computed by PubChem | |
Isomeric SMILES |
CC(C)(C)OC(=O)NCCCC[C@@H](C(=O)N)NC(=O)OCC1=CC=CC=C1 | |
Details | Computed by OEChem 2.3.0 (PubChem release 2021.05.07) | |
Source | PubChem | |
URL | https://pubchem.ncbi.nlm.nih.gov | |
Description | Data deposited in or computed by PubChem | |
Molecular Formula |
C19H29N3O5 | |
Details | Computed by PubChem 2.1 (PubChem release 2021.05.07) | |
Source | PubChem | |
URL | https://pubchem.ncbi.nlm.nih.gov | |
Description | Data deposited in or computed by PubChem | |
Molecular Weight |
379.5 g/mol | |
Details | Computed by PubChem 2.1 (PubChem release 2021.05.07) | |
Source | PubChem | |
URL | https://pubchem.ncbi.nlm.nih.gov | |
Description | Data deposited in or computed by PubChem | |
Disclaimer and Information on In-Vitro Research Products
Please be aware that all articles and product information presented on BenchChem are intended solely for informational purposes. The products available for purchase on BenchChem are specifically designed for in-vitro studies, which are conducted outside of living organisms. In-vitro studies, derived from the Latin term "in glass," involve experiments performed in controlled laboratory settings using cells or tissues. It is important to note that these products are not categorized as medicines or drugs, and they have not received approval from the FDA for the prevention, treatment, or cure of any medical condition, ailment, or disease. We must emphasize that any form of bodily introduction of these products into humans or animals is strictly prohibited by law. It is essential to adhere to these guidelines to ensure compliance with legal and ethical standards in research and experimentation.