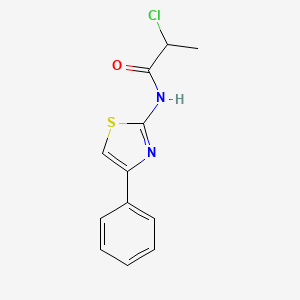
2-chloro-N-(4-phenyl-1,3-thiazol-2-yl)propanamide
Overview
Description
2-chloro-N-(4-phenyl-1,3-thiazol-2-yl)propanamide is a synthetic organic compound that belongs to the class of thiazole derivatives. Thiazoles are heterocyclic compounds containing both sulfur and nitrogen atoms in a five-membered ring. This particular compound is characterized by the presence of a chloro group, a phenyl group, and a propanamide moiety attached to the thiazole ring. Thiazole derivatives are known for their diverse biological activities, including antimicrobial, antifungal, and anticancer properties .
Mechanism of Action
Target of Action
2-chloro-N-(4-phenyl-1,3-thiazol-2-yl)propanamide is a derivative of thiazole, a heterocyclic compound that exhibits a wide range of biological activities Thiazole derivatives have been reported to exhibit antimicrobial, antifungal, anti-inflammatory, and antitumor activities . These activities suggest that the compound may target a variety of enzymes or receptors involved in these biological processes.
Mode of Action
Thiazole derivatives are known to interact with their targets through various mechanisms, depending on the specific substituents on the thiazole ring . These interactions can lead to changes in the activity of the target, which can result in the observed biological effects.
Biochemical Pathways
Given the broad range of biological activities exhibited by thiazole derivatives, it is likely that this compound affects multiple pathways . These could include pathways related to microbial growth, inflammation, and tumor progression, among others.
Pharmacokinetics
Thiazole derivatives are generally slightly soluble in water, soluble in alcohol and ether, and sparingly soluble in organic solvents . These properties can influence the compound’s bioavailability and distribution within the body.
Result of Action
Thiazole derivatives have been reported to exhibit antimicrobial, antifungal, anti-inflammatory, and antitumor activities . These effects suggest that the compound may inhibit the growth of microbes and tumors, reduce inflammation, and other cellular effects related to these activities.
Action Environment
Factors such as ph, temperature, and the presence of other substances can potentially affect the activity and stability of thiazole derivatives .
Biochemical Analysis
Biochemical Properties
It is known that substituents on a particular position of the thiazole ring affect the biological outcomes to a great extent .
Cellular Effects
It is known that thiazoles, a class of compounds to which this compound belongs, exhibit a wide range of biological activities, such as antibacterial, antifungal, anti-inflammatory, antitumor, antitubercular, antidiabetic, antiviral, and antioxidant .
Molecular Mechanism
It is known that the molecular electrostatic potential (MEP) surface of the thiazole ring plays a significant role in drug–target protein interaction .
Temporal Effects in Laboratory Settings
It is known that the inhibitory concentrations of newly synthesized molecules were compared with the standard reference drugs .
Dosage Effects in Animal Models
It is known that the inhibitory concentrations of newly synthesized molecules were compared with the standard reference drugs .
Metabolic Pathways
It is known that substituents on a particular position of the thiazole ring affect the biological outcomes to a great extent .
Transport and Distribution
It is known that the molecular electrostatic potential (MEP) surface of the thiazole ring plays a significant role in drug–target protein interaction .
Subcellular Localization
It is known that the molecular electrostatic potential (MEP) surface of the thiazole ring plays a significant role in drug–target protein interaction .
Preparation Methods
The synthesis of 2-chloro-N-(4-phenyl-1,3-thiazol-2-yl)propanamide typically involves the following steps:
Formation of the Thiazole Ring: The thiazole ring can be synthesized through a cyclization reaction involving a thiourea derivative and an α-haloketone.
Introduction of the Chloro Group: The chloro group can be introduced through a halogenation reaction using reagents like thionyl chloride or phosphorus pentachloride.
Attachment of the Propanamide Moiety: The final step involves the acylation of the thiazole derivative with a suitable acylating agent, such as propanoyl chloride, in the presence of a base like pyridine or triethylamine.
Industrial production methods for this compound may involve optimization of these synthetic routes to achieve higher yields and purity, often using continuous flow reactors and automated synthesis techniques.
Chemical Reactions Analysis
2-chloro-N-(4-phenyl-1,3-thiazol-2-yl)propanamide can undergo various chemical reactions, including:
Substitution Reactions: The chloro group can be substituted with other nucleophiles, such as amines or thiols, to form new derivatives.
Oxidation Reactions: The thiazole ring can be oxidized using oxidizing agents like hydrogen peroxide or m-chloroperbenzoic acid to form sulfoxides or sulfones.
Reduction Reactions: The carbonyl group in the propanamide moiety can be reduced to an alcohol using reducing agents like lithium aluminum hydride or sodium borohydride.
The major products formed from these reactions depend on the specific reagents and conditions used. For example, substitution with an amine can yield an amide derivative, while oxidation of the thiazole ring can produce a sulfone derivative.
Scientific Research Applications
2-chloro-N-(4-phenyl-1,3-thiazol-2-yl)propanamide has several scientific research applications, including:
Comparison with Similar Compounds
2-chloro-N-(4-phenyl-1,3-thiazol-2-yl)propanamide can be compared with other thiazole derivatives, such as:
2-aminothiazole: Known for its anticancer and antimicrobial activities.
4-phenylthiazole: Exhibits antifungal and anti-inflammatory properties.
Benzothiazole derivatives: These compounds have shown significant antitubercular and anticancer activities.
The uniqueness of this compound lies in its specific substitution pattern, which imparts distinct biological activities and chemical reactivity compared to other thiazole derivatives.
Properties
IUPAC Name |
2-chloro-N-(4-phenyl-1,3-thiazol-2-yl)propanamide | |
---|---|---|
Details | Computed by Lexichem TK 2.7.0 (PubChem release 2021.05.07) | |
Source | PubChem | |
URL | https://pubchem.ncbi.nlm.nih.gov | |
Description | Data deposited in or computed by PubChem | |
InChI |
InChI=1S/C12H11ClN2OS/c1-8(13)11(16)15-12-14-10(7-17-12)9-5-3-2-4-6-9/h2-8H,1H3,(H,14,15,16) | |
Details | Computed by InChI 1.0.6 (PubChem release 2021.05.07) | |
Source | PubChem | |
URL | https://pubchem.ncbi.nlm.nih.gov | |
Description | Data deposited in or computed by PubChem | |
InChI Key |
HZWSBFODOBIPRE-UHFFFAOYSA-N | |
Details | Computed by InChI 1.0.6 (PubChem release 2021.05.07) | |
Source | PubChem | |
URL | https://pubchem.ncbi.nlm.nih.gov | |
Description | Data deposited in or computed by PubChem | |
Canonical SMILES |
CC(C(=O)NC1=NC(=CS1)C2=CC=CC=C2)Cl | |
Details | Computed by OEChem 2.3.0 (PubChem release 2021.05.07) | |
Source | PubChem | |
URL | https://pubchem.ncbi.nlm.nih.gov | |
Description | Data deposited in or computed by PubChem | |
Molecular Formula |
C12H11ClN2OS | |
Details | Computed by PubChem 2.1 (PubChem release 2021.05.07) | |
Source | PubChem | |
URL | https://pubchem.ncbi.nlm.nih.gov | |
Description | Data deposited in or computed by PubChem | |
Molecular Weight |
266.75 g/mol | |
Details | Computed by PubChem 2.1 (PubChem release 2021.05.07) | |
Source | PubChem | |
URL | https://pubchem.ncbi.nlm.nih.gov | |
Description | Data deposited in or computed by PubChem | |
Synthesis routes and methods
Procedure details
Disclaimer and Information on In-Vitro Research Products
Please be aware that all articles and product information presented on BenchChem are intended solely for informational purposes. The products available for purchase on BenchChem are specifically designed for in-vitro studies, which are conducted outside of living organisms. In-vitro studies, derived from the Latin term "in glass," involve experiments performed in controlled laboratory settings using cells or tissues. It is important to note that these products are not categorized as medicines or drugs, and they have not received approval from the FDA for the prevention, treatment, or cure of any medical condition, ailment, or disease. We must emphasize that any form of bodily introduction of these products into humans or animals is strictly prohibited by law. It is essential to adhere to these guidelines to ensure compliance with legal and ethical standards in research and experimentation.