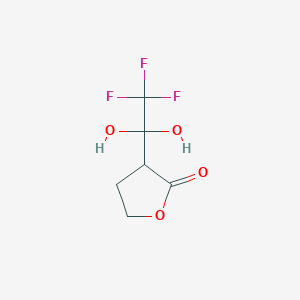
3-(2,2,2-Trifluoro-1,1-dihydroxyethyl)oxolan-2-one
- Click on QUICK INQUIRY to receive a quote from our team of experts.
- With the quality product at a COMPETITIVE price, you can focus more on your research.
Overview
Description
3-(2,2,2-Trifluoro-1,1-dihydroxyethyl)oxolan-2-one is a chemical compound with the molecular formula C6H7F3O4 and a molecular weight of 200.11 g/mol It is known for its unique structure, which includes a trifluoromethyl group and a dihydroxyethyl group attached to an oxolan-2-one ring
Preparation Methods
Synthetic Routes and Reaction Conditions
The synthesis of 3-(2,2,2-Trifluoro-1,1-dihydroxyethyl)oxolan-2-one typically involves the reaction of a trifluoromethyl ketone with an appropriate diol under acidic or basic conditions. One common method involves the use of trifluoroacetone and ethylene glycol in the presence of a catalyst such as p-toluenesulfonic acid. The reaction proceeds through the formation of an intermediate, which then cyclizes to form the oxolan-2-one ring .
Industrial Production Methods
Industrial production of this compound may involve similar synthetic routes but on a larger scale. The reaction conditions are optimized to ensure high yield and purity. The use of continuous flow reactors and advanced purification techniques such as crystallization and chromatography are common in industrial settings to achieve the desired product quality .
Chemical Reactions Analysis
Types of Reactions
3-(2,2,2-Trifluoro-1,1-dihydroxyethyl)oxolan-2-one undergoes various types of chemical reactions, including:
Oxidation: The compound can be oxidized to form corresponding carboxylic acids or ketones.
Reduction: Reduction reactions can convert the oxolan-2-one ring to a more saturated form.
Substitution: The trifluoromethyl group can be substituted with other functional groups under specific conditions.
Common Reagents and Conditions
Oxidation: Common oxidizing agents include potassium permanganate (KMnO4) and chromium trioxide (CrO3).
Reduction: Reducing agents such as lithium aluminum hydride (LiAlH4) and sodium borohydride (NaBH4) are used.
Substitution: Nucleophilic substitution reactions often involve reagents like sodium hydride (NaH) and various alkyl halides.
Major Products
The major products formed from these reactions depend on the specific conditions and reagents used. For example, oxidation may yield trifluoromethyl carboxylic acids, while reduction can produce saturated oxolane derivatives .
Scientific Research Applications
3-(2,2,2-Trifluoro-1,1-dihydroxyethyl)oxolan-2-one has a wide range of applications in scientific research:
Chemistry: It is used as a building block in the synthesis of more complex molecules and as a reagent in various organic reactions.
Biology: The compound is studied for its potential biological activity and interactions with biomolecules.
Medicine: Research is ongoing to explore its potential as a pharmaceutical intermediate or active ingredient.
Mechanism of Action
The mechanism of action of 3-(2,2,2-Trifluoro-1,1-dihydroxyethyl)oxolan-2-one involves its interaction with specific molecular targets and pathways. The trifluoromethyl group is known to enhance the compound’s lipophilicity and metabolic stability, which can affect its biological activity. The dihydroxyethyl group may participate in hydrogen bonding and other interactions with target molecules, influencing the compound’s overall efficacy .
Comparison with Similar Compounds
Similar Compounds
- 3-(2,2,2-Trifluoro-1-hydroxyethyl)oxolan-2-one
- 3-(2,2,2-Trifluoroethyl)oxolan-2-one
- 3-(2,2,2-Trifluoro-1,1-dihydroxypropyl)oxolan-2-one
Uniqueness
3-(2,2,2-Trifluoro-1,1-dihydroxyethyl)oxolan-2-one is unique due to the presence of both trifluoromethyl and dihydroxyethyl groups, which impart distinct chemical and physical properties. These features make it a valuable compound for various applications, distinguishing it from other similar compounds .
Properties
IUPAC Name |
3-(2,2,2-trifluoro-1,1-dihydroxyethyl)oxolan-2-one |
Source
|
---|---|---|
Details | Computed by LexiChem 2.6.6 (PubChem release 2019.06.18) | |
Source | PubChem | |
URL | https://pubchem.ncbi.nlm.nih.gov | |
Description | Data deposited in or computed by PubChem | |
InChI |
InChI=1S/C6H7F3O4/c7-6(8,9)5(11,12)3-1-2-13-4(3)10/h3,11-12H,1-2H2 |
Source
|
Details | Computed by InChI 1.0.5 (PubChem release 2019.06.18) | |
Source | PubChem | |
URL | https://pubchem.ncbi.nlm.nih.gov | |
Description | Data deposited in or computed by PubChem | |
InChI Key |
UVUNIQLSVFYTIM-UHFFFAOYSA-N |
Source
|
Details | Computed by InChI 1.0.5 (PubChem release 2019.06.18) | |
Source | PubChem | |
URL | https://pubchem.ncbi.nlm.nih.gov | |
Description | Data deposited in or computed by PubChem | |
Canonical SMILES |
C1COC(=O)C1C(C(F)(F)F)(O)O |
Source
|
Details | Computed by OEChem 2.1.5 (PubChem release 2019.06.18) | |
Source | PubChem | |
URL | https://pubchem.ncbi.nlm.nih.gov | |
Description | Data deposited in or computed by PubChem | |
Molecular Formula |
C6H7F3O4 |
Source
|
Details | Computed by PubChem 2.1 (PubChem release 2019.06.18) | |
Source | PubChem | |
URL | https://pubchem.ncbi.nlm.nih.gov | |
Description | Data deposited in or computed by PubChem | |
Molecular Weight |
200.11 g/mol |
Source
|
Details | Computed by PubChem 2.1 (PubChem release 2021.05.07) | |
Source | PubChem | |
URL | https://pubchem.ncbi.nlm.nih.gov | |
Description | Data deposited in or computed by PubChem | |
Disclaimer and Information on In-Vitro Research Products
Please be aware that all articles and product information presented on BenchChem are intended solely for informational purposes. The products available for purchase on BenchChem are specifically designed for in-vitro studies, which are conducted outside of living organisms. In-vitro studies, derived from the Latin term "in glass," involve experiments performed in controlled laboratory settings using cells or tissues. It is important to note that these products are not categorized as medicines or drugs, and they have not received approval from the FDA for the prevention, treatment, or cure of any medical condition, ailment, or disease. We must emphasize that any form of bodily introduction of these products into humans or animals is strictly prohibited by law. It is essential to adhere to these guidelines to ensure compliance with legal and ethical standards in research and experimentation.