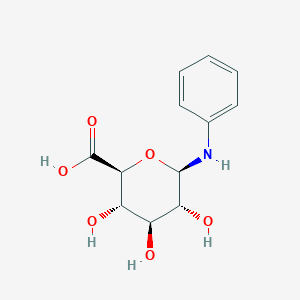
Aniline beta-D-Glucuronide
Overview
Description
DL-Menthol is a naturally occurring compound primarily derived from aromatic plants, particularly through the steam distillation of cornmint oil. It belongs to the class of monoterpenes, which are organic compounds found in essential oils. DL-Menthol is known for its cooling sensation and medicinal properties, making it a versatile compound used in various industries such as pharmaceuticals, cosmetics, and food .
Preparation Methods
Synthetic Routes and Reaction Conditions: DL-Menthol can be synthesized through several methods. One common synthetic route involves the hydrogenation of thymol or pulegone. The hydrogenation process typically uses a metal catalyst such as palladium or nickel under high pressure and temperature conditions .
Industrial Production Methods: Industrial production of DL-Menthol often involves the crystallization of menthol from peppermint oil or other mint oils. The process includes steam distillation of the oil, followed by cooling and crystallization to separate menthol crystals. Another method involves the asymmetric hydrogenation of citral, which is then converted into menthol through further synthesis steps .
Chemical Reactions Analysis
Types of Reactions: DL-Menthol undergoes various chemical reactions, including:
Oxidation: DL-Menthol can be oxidized to menthone using oxidizing agents such as potassium permanganate or chromic acid.
Reduction: Reduction of menthone can yield DL-Menthol using reducing agents like sodium borohydride.
Substitution: DL-Menthol can undergo substitution reactions, such as esterification with acetic anhydride to form menthyl acetate.
Common Reagents and Conditions:
Oxidation: Potassium permanganate, chromic acid.
Reduction: Sodium borohydride, lithium aluminum hydride.
Substitution: Acetic anhydride, sulfuric acid.
Major Products:
Oxidation: Menthone.
Reduction: DL-Menthol.
Substitution: Menthyl acetate.
Scientific Research Applications
DL-Menthol has a wide range of scientific research applications:
Mechanism of Action
DL-Menthol primarily activates the cold-sensitive TRPM8 receptors in the skin. Upon topical application, it causes a feeling of coolness by stimulating ‘cold’ receptors and inhibiting calcium ion currents in neuronal membranes. Additionally, DL-Menthol may exert analgesic properties through kappa-opioid receptor agonism .
Comparison with Similar Compounds
D-Menthol: The other enantiomer of menthol, which is less commonly used due to its lower availability.
Isomenthol: An isomer of menthol with similar cooling properties but different sensory characteristics.
Uniqueness of DL-Menthol: DL-Menthol is a racemic mixture of D- and L-menthol, providing a balanced cooling effect and making it suitable for various applications where a moderate cooling sensation is desired. Its versatility and availability make it a valuable compound in multiple industries .
Biological Activity
Aniline beta-D-glucuronide (ABG) is a significant metabolite formed from aniline, an aromatic amine widely used in various industrial applications, including dye production and pharmaceuticals. Understanding the biological activity of ABG is crucial for assessing its pharmacokinetics, toxicity, and potential therapeutic applications.
Chemical Structure and Formation
ABG is synthesized through glucuronidation , a metabolic process where aniline conjugates with glucuronic acid, primarily catalyzed by UDP-glucuronosyltransferases (UGTs) in the liver. The molecular formula for ABG is , with a molecular weight of approximately 269.25 g/mol. This conjugation enhances the solubility of aniline, facilitating its excretion via renal pathways and reducing its potential toxicity.
Detoxification Mechanism
The formation of ABG plays a critical role in detoxifying aniline. By converting aniline into a more water-soluble form, ABG minimizes the harmful effects associated with aniline exposure. This metabolic pathway is essential for reducing risks related to chronic exposure to aniline, which has been linked to various health issues, including carcinogenicity and hematotoxicity .
Interaction with Other Compounds
Research indicates that ABG can influence the bioavailability and toxicity of other drugs when co-administered. It may affect pharmacokinetics by competing for glucuronidation enzymes or altering metabolic pathways of concomitant medications. This interaction underscores the importance of understanding ABG's role in drug metabolism and potential drug-drug interactions.
Carcinogenic Potential
Studies have shown that chronic exposure to aniline can lead to adverse health effects, including bladder tumors among workers exposed to aniline dyes. However, the specific role of ABG in this context remains under investigation. While some studies report that high doses of aniline can induce genetic damage and mutagenic effects, the exact relationship between ABG and these outcomes requires further exploration .
Genotoxicity Assessments
Genotoxicity studies involving aniline have yielded mixed results. For instance, while some tests indicate that aniline can induce chromosomal aberrations in certain animal models, others have shown no significant DNA damage at non-toxic concentrations. These findings highlight the need for more comprehensive studies to elucidate the genotoxic potential of ABG itself .
Case Studies and Research Findings
Therapeutic Applications
Emerging research suggests that glucuronides like ABG may have therapeutic implications beyond detoxification. For example, β-glucuronidase (βG), which hydrolyzes glucuronides back to their active forms, is being explored as a potential target for cancer therapy due to its elevated expression in tumor microenvironments. This mechanism could enable selective drug activation at tumor sites, enhancing therapeutic efficacy while minimizing systemic toxicity .
Properties
IUPAC Name |
(2S,3S,4S,5R,6R)-6-anilino-3,4,5-trihydroxyoxane-2-carboxylic acid | |
---|---|---|
Source | PubChem | |
URL | https://pubchem.ncbi.nlm.nih.gov | |
Description | Data deposited in or computed by PubChem | |
InChI |
InChI=1S/C12H15NO6/c14-7-8(15)10(12(17)18)19-11(9(7)16)13-6-4-2-1-3-5-6/h1-5,7-11,13-16H,(H,17,18)/t7-,8-,9+,10-,11+/m0/s1 | |
Source | PubChem | |
URL | https://pubchem.ncbi.nlm.nih.gov | |
Description | Data deposited in or computed by PubChem | |
InChI Key |
SPTXZDAXCOTKFR-QUARPLMYSA-N | |
Source | PubChem | |
URL | https://pubchem.ncbi.nlm.nih.gov | |
Description | Data deposited in or computed by PubChem | |
Canonical SMILES |
C1=CC=C(C=C1)NC2C(C(C(C(O2)C(=O)O)O)O)O | |
Source | PubChem | |
URL | https://pubchem.ncbi.nlm.nih.gov | |
Description | Data deposited in or computed by PubChem | |
Isomeric SMILES |
C1=CC=C(C=C1)N[C@H]2[C@@H]([C@H]([C@@H]([C@H](O2)C(=O)O)O)O)O | |
Source | PubChem | |
URL | https://pubchem.ncbi.nlm.nih.gov | |
Description | Data deposited in or computed by PubChem | |
Molecular Formula |
C12H15NO6 | |
Source | PubChem | |
URL | https://pubchem.ncbi.nlm.nih.gov | |
Description | Data deposited in or computed by PubChem | |
DSSTOX Substance ID |
DTXSID20435012 | |
Record name | N-Phenyl-beta-D-glucopyranuronosylamine | |
Source | EPA DSSTox | |
URL | https://comptox.epa.gov/dashboard/DTXSID20435012 | |
Description | DSSTox provides a high quality public chemistry resource for supporting improved predictive toxicology. | |
Molecular Weight |
269.25 g/mol | |
Source | PubChem | |
URL | https://pubchem.ncbi.nlm.nih.gov | |
Description | Data deposited in or computed by PubChem | |
CAS No. |
92117-30-1 | |
Record name | N-Phenyl-beta-D-glucopyranuronosylamine | |
Source | EPA DSSTox | |
URL | https://comptox.epa.gov/dashboard/DTXSID20435012 | |
Description | DSSTox provides a high quality public chemistry resource for supporting improved predictive toxicology. | |
Retrosynthesis Analysis
AI-Powered Synthesis Planning: Our tool employs the Template_relevance Pistachio, Template_relevance Bkms_metabolic, Template_relevance Pistachio_ringbreaker, Template_relevance Reaxys, Template_relevance Reaxys_biocatalysis model, leveraging a vast database of chemical reactions to predict feasible synthetic routes.
One-Step Synthesis Focus: Specifically designed for one-step synthesis, it provides concise and direct routes for your target compounds, streamlining the synthesis process.
Accurate Predictions: Utilizing the extensive PISTACHIO, BKMS_METABOLIC, PISTACHIO_RINGBREAKER, REAXYS, REAXYS_BIOCATALYSIS database, our tool offers high-accuracy predictions, reflecting the latest in chemical research and data.
Strategy Settings
Precursor scoring | Relevance Heuristic |
---|---|
Min. plausibility | 0.01 |
Model | Template_relevance |
Template Set | Pistachio/Bkms_metabolic/Pistachio_ringbreaker/Reaxys/Reaxys_biocatalysis |
Top-N result to add to graph | 6 |
Feasible Synthetic Routes
Disclaimer and Information on In-Vitro Research Products
Please be aware that all articles and product information presented on BenchChem are intended solely for informational purposes. The products available for purchase on BenchChem are specifically designed for in-vitro studies, which are conducted outside of living organisms. In-vitro studies, derived from the Latin term "in glass," involve experiments performed in controlled laboratory settings using cells or tissues. It is important to note that these products are not categorized as medicines or drugs, and they have not received approval from the FDA for the prevention, treatment, or cure of any medical condition, ailment, or disease. We must emphasize that any form of bodily introduction of these products into humans or animals is strictly prohibited by law. It is essential to adhere to these guidelines to ensure compliance with legal and ethical standards in research and experimentation.