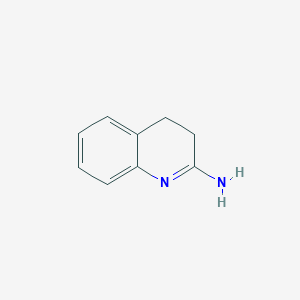
3,4-Dihydroquinolin-2-amine
Overview
Description
3,4-Dihydroquinolin-2-amine is a partially hydrogenated quinoline derivative characterized by a six-membered aromatic ring fused to a saturated dihydroquinoline core. This scaffold is widely studied in medicinal chemistry due to its versatility as a precursor for bioactive molecules, including antiviral agents and kinase inhibitors . Key synthetic routes involve reductive amination, nucleophilic substitution, and catalytic hydrogenation, as demonstrated in the preparation of derivatives such as 6-amino-1-(2-(dimethylamino)ethyl)-3,4-dihydroquinolin-2(1H)-one (compound 20) and its reduced analogs (e.g., compound 32) .
Preparation Methods
Synthetic Routes and Reaction Conditions
The synthesis of 3,4-Dihydro-1H-quinolin-(2E)-ylideneamine can be achieved through various synthetic routes. One common method involves the reaction of 2-alkynylanilines with ketones in the presence of Brønsted acid or Lewis acid catalysts . For example, the reaction of 2-ethynylanilines with ketones in the presence of p-toluenesulfonic acid monohydrate in ethanol at reflux conditions yields the desired quinoline derivatives . Another approach involves the use of FeCl3 as a catalyst in toluene at elevated temperatures .
Industrial Production Methods
Industrial production of 3,4-Dihydro-1H-quinolin-(2E)-ylideneamine typically involves large-scale synthesis using optimized reaction conditions to ensure high yield and purity. The choice of catalysts, solvents, and reaction parameters is crucial for efficient production. Continuous flow reactors and automated synthesis platforms are often employed to streamline the production process and minimize waste.
Chemical Reactions Analysis
Types of Reactions
3,4-Dihydro-1H-quinolin-(2E)-ylideneamine undergoes various chemical reactions, including:
Oxidation: The compound can be oxidized to form quinoline-2-one derivatives.
Reduction: Reduction reactions can convert the ylideneamine group to an amine.
Substitution: Electrophilic and nucleophilic substitution reactions can introduce different functional groups onto the quinoline core.
Common Reagents and Conditions
Oxidation: Common oxidizing agents include potassium permanganate and hydrogen peroxide.
Reduction: Reducing agents such as sodium borohydride and lithium aluminum hydride are used.
Substitution: Reagents like halogens, alkyl halides, and organometallic compounds are employed for substitution reactions.
Major Products
The major products formed from these reactions include various substituted quinoline derivatives, which can exhibit different biological and chemical properties depending on the nature of the substituents.
Scientific Research Applications
1.1. Neuropharmacology
3,4-Dihydroquinolin-2-amine derivatives have been synthesized and evaluated for their potential as dopamine receptor D2 modulators. A study revealed that certain derivatives exhibited high affinity for D2 receptors and showed promise in treating central nervous system disorders such as schizophrenia. The compound 5e demonstrated a favorable profile for crossing the blood-brain barrier while maintaining low cytotoxicity, indicating its potential for neuropharmacological applications .
1.2. Matrix Metalloproteinase Inhibition
Research has identified 3,4-dihydroquinolin-2-one derivatives as inhibitors of matrix metalloproteinases (MMPs). These enzymes are implicated in various diseases characterized by tissue breakdown, including heart disease and arthritis. The inhibition of MMPs by these compounds suggests their utility in treating conditions such as cardiac insufficiency and inflammatory bowel disease .
Synthesis and Structural Variations
The synthesis of this compound can be achieved through various methods, including redox-triggered reactions and asymmetric synthesis techniques. Recent advancements have allowed for the development of chiral variants with complex structures, enhancing their potential biological activities .
3.1. Enzyme Inhibition
Several studies have reported the enzyme inhibition properties of this compound derivatives. For instance, peptide-dihydroquinolinone conjugates have shown inhibitory effects against human carbonic anhydrase II (hCA II), with IC50 values ranging from 15.7 to 65.7 µM . This suggests potential applications in treating conditions related to carbonic anhydrase activity.
3.2. Antioxidant Properties
The antioxidant activities of some this compound derivatives have been evaluated using DPPH radical scavenging assays. Although these compounds displayed weaker antioxidant properties compared to standard antioxidants like α-tocopherol, their structural modifications could enhance these activities in future studies .
4.1. Case Study: Neuropharmacological Potential
A pilot study focused on synthesizing new derivatives based on the approved drug aripiprazole highlighted the need for developing effective neuroleptic drugs targeting D2 receptors . The findings indicated that the synthesized compounds could serve as potential alternatives or adjuncts to existing treatments for schizophrenia.
4.2. Case Study: MMP Inhibition
Another significant study investigated the efficacy of 3,4-dihydroquinolin-2-one derivatives as MMP inhibitors, demonstrating their potential in managing diseases associated with extracellular matrix degradation . This case study underscores the relevance of these compounds in therapeutic strategies against chronic diseases.
Summary Table of Applications
Mechanism of Action
The mechanism of action of 3,4-Dihydro-1H-quinolin-(2E)-ylideneamine involves its interaction with specific molecular targets and pathways. For example, it acts as an inhibitor of aldosterone synthase (CYP11B2), which catalyzes the conversion of 11-deoxycorticosterone to aldosterone . By inhibiting this enzyme, the compound can potentially reduce elevated aldosterone levels associated with hypertension and heart failure.
Comparison with Similar Compounds
Structural and Functional Comparison with Similar Compounds
1,2,3,4-Tetrahydroquinoline Derivatives
Fully saturated 1,2,3,4-tetrahydroquinoline analogs exhibit enhanced conformational flexibility compared to 3,4-dihydroquinolin-2-amine. For example:
- 1-(2-(Diethylamino)ethyl)-1,2,3,4-tetrahydroquinolin-6-amine (47): This compound, synthesized via LiAlH4 reduction of a dihydroquinolinone precursor, shows a yield of 90% and distinct NMR signals (δ 1.08 ppm for diethyl groups) .
- 1-(2-(Dimethylamino)ethyl)-8-fluoro-1,2,3,4-tetrahydroquinolin-6-amine (48): Incorporation of a fluorine substituent at position 8 enhances polarity, reflected in its ESI-MS data (m/z 238) .
Key Differences :
Substituted Quinoline Amines
Trifluoromethyl-Substituted Quinolines
Compounds like 2-(trifluoromethyl)quinolin-4-amine (CAS 1700-93-2) and its positional isomers (e.g., 7-amine, CAS 2241594-48-7) exhibit enhanced metabolic stability due to the electron-withdrawing CF3 group. These analogs are structurally distinct from this compound but share applications in drug discovery .
Imidazo[4,5-f]quinolines
3,4-Dimethyl-1,3-dihydro-2H-imidazo[4,5-f]quinolin-2-imine () features a fused imidazole ring, conferring planar rigidity. This contrasts with the flexible dihydroquinoline core, impacting binding affinity to nucleic acids or enzymes .
Dihydroquinoxalin-2-amine Derivatives
3,4-Dihydroquinoxalin-2-amine derivatives (e.g., spirocyclic analogs synthesized via three-component reactions) differ in their bicyclic nitrogen-containing core. These compounds are prioritized for antimicrobial applications, whereas dihydroquinolines are more commonly explored in CNS and antiviral research .
Spectroscopic Profiles
Compound ID | 1H NMR (δ, ppm) | MS (m/z) | |
---|---|---|---|
47 | 1.08 (t, J = 7.2 Hz, 6H, CH2CH3) | 248.2 (M + 1) | |
33 | 2.30 (s, 3H, N-CH3) | 260.2 (M + 1) | |
26 | N/A (thiophene-carboximidamide adduct) | 56% yield (no MS) |
Biological Activity
3,4-Dihydroquinolin-2-amine, a compound belonging to the dihydroquinoline class, exhibits a range of biological activities that have garnered attention in medicinal chemistry. This article explores its biological activity, focusing on its mechanisms of action, potential therapeutic applications, and relevant research findings.
Chemical Structure and Properties
This compound is characterized by a six-membered nitrogen-containing heterocyclic structure. Its unique chemical properties arise from the presence of both an amino group and a carbonyl group within the quinoline framework, making it a versatile scaffold for drug development.
The biological activity of this compound is primarily attributed to its interactions with various molecular targets:
- Dopamine Receptor Modulation : Recent studies have synthesized derivatives of 3,4-dihydroquinolin-2(1H)-one as potential modulators of dopamine receptor D2 (D2R). One notable compound demonstrated high affinity for D2R and showed promise for treating central nervous system disorders such as schizophrenia .
- Cholinesterase Inhibition : Compounds derived from this scaffold have been evaluated for their inhibitory effects on acetylcholinesterase (AChE) and monoamine oxidases (MAOs). For instance, one derivative exhibited IC50 values of 0.28 µM for AChE and 0.91 µM for hMAO-A, indicating significant potential for treating Alzheimer's disease .
Case Studies and Experimental Results
- Dopamine Receptor D2 Modulators :
- Acetylcholinesterase Inhibition :
- Anticancer Activity :
Data Table: Biological Activities Summary
Compound | Target/Activity | IC50 Value (µM) | Notes |
---|---|---|---|
5e | D2R Affinity | N/A | High affinity but unique binding mode |
3e | AChE Inhibition | 0.28 (eeAChE) | Effective inhibitor with low cytotoxicity |
MAO-A Inhibition | 0.91 | Balanced inhibition profile | |
MAO-B Inhibition | 2.81 | ||
Various Tetrahydroquinolines | Antiproliferative Activity | N/A | Effective against DU145 and MCF7 cell lines |
Q & A
Basic Research Questions
Q. What are the key considerations for synthesizing 3,4-Dihydroquinolin-2-amine derivatives with high yield?
- Methodological Answer : Optimize reduction steps using agents like LiAlH₄ to reduce 3,4-dihydroquinolin-2(1H)-ones to the corresponding amines. Control reaction time (e.g., 24 hours at room temperature) and stoichiometry (e.g., 4:1 molar ratio of LiAlH₄ to substrate) to minimize side products . For enantioselective synthesis, employ radical cyclization strategies with chiral catalysts to achieve high enantiomeric excess (e.g., 6-exo-trig cyclizations) .
Q. How can researchers characterize this compound derivatives effectively?
- Methodological Answer : Use a combination of ¹H NMR (CDCl₃ or DMSO-d₆) to identify proton environments (e.g., aromatic protons at δ 6.3–6.6 ppm, methylene/methyl groups at δ 1.4–3.3 ppm) and mass spectrometry (ESI-MS) to confirm molecular ions (e.g., [M + H⁺] peaks at m/z 238–260) . Purity can be assessed via HPLC with UV detection at 254 nm.
Q. What biological assays are suitable for preliminary evaluation of this compound derivatives?
- Methodological Answer : Screen for antimicrobial activity using MIC (Minimum Inhibitory Concentration) assays against Gram-positive/negative bacteria. For anticancer potential, employ MTT assays on cancer cell lines (e.g., HeLa, MCF-7) and compare IC₅₀ values. Include positive controls (e.g., doxorubicin) and validate results with triplicate experiments .
Advanced Research Questions
Q. How can structural modifications of this compound enhance target selectivity in neurological disorders?
- Methodological Answer : Introduce substituents like trifluoromethyl groups or fluorinated aryl rings to improve blood-brain barrier penetration. Evaluate binding affinity to serotonin/dopamine receptors via radioligand assays (e.g., ³H-labeled ligands). Use molecular docking to predict interactions with active sites (e.g., 5-HT₂A receptors) .
Q. What strategies resolve contradictions in biological activity data across derivatives?
- Methodological Answer : Reassess compound purity (HPLC, NMR), confirm stereochemistry (X-ray crystallography), and standardize assay conditions (e.g., cell line passage number, serum concentration). Cross-validate using orthogonal assays (e.g., flow cytometry for apoptosis vs. Western blot for caspase activation) .
Q. How can enantioselective synthesis of this compound derivatives be optimized for scalability?
- Methodological Answer : Employ asymmetric hydrogenation with chiral phosphine ligands (e.g., BINAP) or organocatalytic methods (e.g., proline derivatives) to achieve >90% ee. Monitor reaction progress via chiral HPLC and optimize solvent systems (e.g., THF/MeOH) for large-scale reductions .
Q. What are the limitations of current SAR studies on this compound derivatives?
Properties
IUPAC Name |
3,4-dihydroquinolin-2-amine | |
---|---|---|
Details | Computed by LexiChem 2.6.6 (PubChem release 2019.06.18) | |
Source | PubChem | |
URL | https://pubchem.ncbi.nlm.nih.gov | |
Description | Data deposited in or computed by PubChem | |
InChI |
InChI=1S/C9H10N2/c10-9-6-5-7-3-1-2-4-8(7)11-9/h1-4H,5-6H2,(H2,10,11) | |
Details | Computed by InChI 1.0.5 (PubChem release 2019.06.18) | |
Source | PubChem | |
URL | https://pubchem.ncbi.nlm.nih.gov | |
Description | Data deposited in or computed by PubChem | |
InChI Key |
RREBANHDFAXBEZ-UHFFFAOYSA-N | |
Details | Computed by InChI 1.0.5 (PubChem release 2019.06.18) | |
Source | PubChem | |
URL | https://pubchem.ncbi.nlm.nih.gov | |
Description | Data deposited in or computed by PubChem | |
Canonical SMILES |
C1CC(=NC2=CC=CC=C21)N | |
Details | Computed by OEChem 2.1.5 (PubChem release 2019.06.18) | |
Source | PubChem | |
URL | https://pubchem.ncbi.nlm.nih.gov | |
Description | Data deposited in or computed by PubChem | |
Molecular Formula |
C9H10N2 | |
Details | Computed by PubChem 2.1 (PubChem release 2019.06.18) | |
Source | PubChem | |
URL | https://pubchem.ncbi.nlm.nih.gov | |
Description | Data deposited in or computed by PubChem | |
Molecular Weight |
146.19 g/mol | |
Details | Computed by PubChem 2.1 (PubChem release 2021.05.07) | |
Source | PubChem | |
URL | https://pubchem.ncbi.nlm.nih.gov | |
Description | Data deposited in or computed by PubChem | |
Synthesis routes and methods
Procedure details
Disclaimer and Information on In-Vitro Research Products
Please be aware that all articles and product information presented on BenchChem are intended solely for informational purposes. The products available for purchase on BenchChem are specifically designed for in-vitro studies, which are conducted outside of living organisms. In-vitro studies, derived from the Latin term "in glass," involve experiments performed in controlled laboratory settings using cells or tissues. It is important to note that these products are not categorized as medicines or drugs, and they have not received approval from the FDA for the prevention, treatment, or cure of any medical condition, ailment, or disease. We must emphasize that any form of bodily introduction of these products into humans or animals is strictly prohibited by law. It is essential to adhere to these guidelines to ensure compliance with legal and ethical standards in research and experimentation.