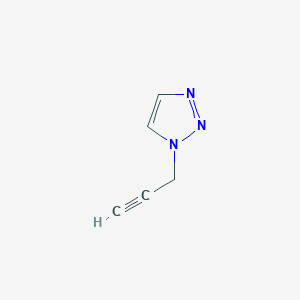
1-(prop-2-yn-1-yl)-1H-1,2,3-triazole
- Click on QUICK INQUIRY to receive a quote from our team of experts.
- With the quality product at a COMPETITIVE price, you can focus more on your research.
Overview
Description
1-(prop-2-yn-1-yl)-1H-1,2,3-triazole is a heterocyclic compound that belongs to the class of 1,2,3-triazoles. This compound is characterized by the presence of a triazole ring, which is a five-membered ring containing three nitrogen atoms and two carbon atoms. The prop-2-yn-1-yl group is attached to the nitrogen atom at position 1 of the triazole ring. This compound is of significant interest in various fields of chemistry due to its unique structural features and reactivity.
Preparation Methods
Synthetic Routes and Reaction Conditions
1-(prop-2-yn-1-yl)-1H-1,2,3-triazole can be synthesized through various methods. One common method involves the cycloaddition reaction between an azide and an alkyne, known as the Huisgen cycloaddition or “click chemistry.” This reaction typically requires a copper(I) catalyst and proceeds under mild conditions to yield the desired triazole compound .
Industrial Production Methods
In an industrial setting, the synthesis of this compound can be scaled up using continuous flow reactors. These reactors allow for precise control of reaction conditions, such as temperature and pressure, and can handle large volumes of reactants. The use of continuous flow reactors also enhances the safety and efficiency of the synthesis process .
Chemical Reactions Analysis
Types of Reactions
1-(prop-2-yn-1-yl)-1H-1,2,3-triazole undergoes various chemical reactions, including:
Oxidation: The compound can be oxidized to form corresponding oxides.
Reduction: Reduction reactions can convert the triazole ring to other nitrogen-containing heterocycles.
Substitution: The triazole ring can undergo substitution reactions with various electrophiles and nucleophiles.
Common Reagents and Conditions
Oxidation: Common oxidizing agents include hydrogen peroxide and potassium permanganate.
Reduction: Reducing agents such as lithium aluminum hydride and sodium borohydride are used.
Substitution: Electrophiles like alkyl halides and nucleophiles like amines are commonly employed.
Major Products Formed
The major products formed from these reactions depend on the specific reagents and conditions used. For example, oxidation may yield triazole oxides, while substitution reactions can produce a variety of substituted triazoles .
Scientific Research Applications
1-(prop-2-yn-1-yl)-1H-1,2,3-triazole has numerous applications in scientific research:
Chemistry: It is used as a building block in the synthesis of more complex molecules, including pharmaceuticals and agrochemicals.
Biology: The compound is studied for its potential biological activities, such as antimicrobial and anticancer properties.
Medicine: It serves as a scaffold for drug development, particularly in the design of enzyme inhibitors and receptor modulators.
Mechanism of Action
The mechanism of action of 1-(prop-2-yn-1-yl)-1H-1,2,3-triazole depends on its specific application. In biological systems, the compound may interact with enzymes or receptors, inhibiting their activity or modulating their function. The triazole ring can form hydrogen bonds and π-π interactions with target molecules, contributing to its binding affinity and specificity .
Comparison with Similar Compounds
Similar Compounds
1-(prop-2-yn-1-yl)-1H-1,2,4-triazole: Similar structure but with a different arrangement of nitrogen atoms in the ring.
1-(prop-2-yn-1-yl)-1H-1,2,3-benzotriazole: Contains a fused benzene ring, leading to different chemical properties.
1-(prop-2-yn-1-yl)-1H-1,2,3-tetrazole: Contains an additional nitrogen atom in the ring, altering its reactivity.
Uniqueness
1-(prop-2-yn-1-yl)-1H-1,2,3-triazole is unique due to its specific arrangement of nitrogen atoms in the triazole ring, which imparts distinct chemical and biological properties. Its ability to undergo a wide range of chemical reactions and its versatility as a building block in synthesis make it a valuable compound in various fields of research .
Properties
IUPAC Name |
1-prop-2-ynyltriazole |
Source
|
---|---|---|
Details | Computed by LexiChem 2.6.6 (PubChem release 2019.06.18) | |
Source | PubChem | |
URL | https://pubchem.ncbi.nlm.nih.gov | |
Description | Data deposited in or computed by PubChem | |
InChI |
InChI=1S/C5H5N3/c1-2-4-8-5-3-6-7-8/h1,3,5H,4H2 |
Source
|
Details | Computed by InChI 1.0.5 (PubChem release 2019.06.18) | |
Source | PubChem | |
URL | https://pubchem.ncbi.nlm.nih.gov | |
Description | Data deposited in or computed by PubChem | |
InChI Key |
BBMDEASYWHQDKZ-UHFFFAOYSA-N |
Source
|
Details | Computed by InChI 1.0.5 (PubChem release 2019.06.18) | |
Source | PubChem | |
URL | https://pubchem.ncbi.nlm.nih.gov | |
Description | Data deposited in or computed by PubChem | |
Canonical SMILES |
C#CCN1C=CN=N1 |
Source
|
Details | Computed by OEChem 2.1.5 (PubChem release 2019.06.18) | |
Source | PubChem | |
URL | https://pubchem.ncbi.nlm.nih.gov | |
Description | Data deposited in or computed by PubChem | |
Molecular Formula |
C5H5N3 |
Source
|
Details | Computed by PubChem 2.1 (PubChem release 2019.06.18) | |
Source | PubChem | |
URL | https://pubchem.ncbi.nlm.nih.gov | |
Description | Data deposited in or computed by PubChem | |
Molecular Weight |
107.11 g/mol |
Source
|
Details | Computed by PubChem 2.1 (PubChem release 2021.05.07) | |
Source | PubChem | |
URL | https://pubchem.ncbi.nlm.nih.gov | |
Description | Data deposited in or computed by PubChem | |
Disclaimer and Information on In-Vitro Research Products
Please be aware that all articles and product information presented on BenchChem are intended solely for informational purposes. The products available for purchase on BenchChem are specifically designed for in-vitro studies, which are conducted outside of living organisms. In-vitro studies, derived from the Latin term "in glass," involve experiments performed in controlled laboratory settings using cells or tissues. It is important to note that these products are not categorized as medicines or drugs, and they have not received approval from the FDA for the prevention, treatment, or cure of any medical condition, ailment, or disease. We must emphasize that any form of bodily introduction of these products into humans or animals is strictly prohibited by law. It is essential to adhere to these guidelines to ensure compliance with legal and ethical standards in research and experimentation.