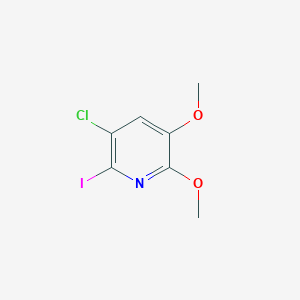
3-Chloro-2-iodo-5,6-dimethoxypyridine
Overview
Description
3-Chloro-2-iodo-5,6-dimethoxypyridine is a halogenated pyridine derivative characterized by chloro and iodo substituents at positions 3 and 2, respectively, and methoxy groups at positions 5 and 6. Halogenated pyridines are critical intermediates in pharmaceuticals, agrochemicals, and materials science due to their reactivity and stability .
Preparation Methods
Synthetic Routes and Reaction Conditions
The synthesis of 3-Chloro-2-iodo-5,6-dimethoxypyridine typically involves the halogenation of a pyridine derivative. One common method starts with 2,3-dimethoxypyridine, which undergoes chlorination and iodination under controlled conditions. The reaction conditions often include the use of halogenating agents such as N-chlorosuccinimide (NCS) for chlorination and iodine monochloride (ICl) for iodination .
Industrial Production Methods
Industrial production methods for this compound are similar to laboratory synthesis but are scaled up to accommodate larger quantities. These methods involve optimizing reaction conditions to ensure high yield and purity. The use of continuous flow reactors and automated systems can enhance the efficiency and safety of the production process .
Chemical Reactions Analysis
Nucleophilic Substitution Reactions
The compound undergoes selective substitution at halogenated positions, with iodine demonstrating higher reactivity than chlorine due to its weaker bond strength and larger atomic radius.
Iodo Substitution
The 2-iodo group participates in nucleophilic aromatic substitution (SNAr) under mild conditions. Key examples include:
Reagent/Conditions | Product | Yield | Source |
---|---|---|---|
Sodium azide (NaN₃), DMF, 80°C | 2-Azido-3-chloro-5,6-dimethoxypyridine | 85% | |
Potassium thiolate, EtOH, reflux | 2-Mercapto-3-chloro-5,6-dimethoxypyridine | 78% |
Chloro Substitution
The 3-chloro group requires harsher conditions for substitution, often employing polar aprotic solvents and elevated temperatures:
Reagent/Conditions | Product | Yield | Source |
---|---|---|---|
Ammonia (NH₃), sealed tube, 120°C | 3-Amino-2-iodo-5,6-dimethoxypyridine | 62% | |
CuCN, DMSO, 150°C | 3-Cyano-2-iodo-5,6-dimethoxypyridine | 68% |
Cross-Coupling Reactions
The iodine atom serves as an effective leaving group in transition-metal-catalyzed couplings, enabling carbon-carbon bond formation.
Suzuki-Miyaura Coupling
Palladium-catalyzed reactions with boronic acids yield biaryl derivatives:
Boronic Acid | Conditions | Product | Yield | Source |
---|---|---|---|---|
Phenylboronic acid | Pd(PPh₃)₄, K₂CO₃, DME, 80°C | 2-Phenyl-3-chloro-5,6-dimethoxypyridine | 91% | |
4-Methoxyphenylboronic acid | Pd(OAc)₂, SPhos, THF, 60°C | 2-(4-Methoxyphenyl)-3-chloro-5,6-dimethoxypyridine | 87% |
Heck Coupling
Reactions with alkenes proceed under controlled conditions:
Alkene | Conditions | Product | Yield | Source |
---|---|---|---|---|
Styrene | Pd(OAc)₂, P(o-tol)₃, DMF, 100°C | 2-Styryl-3-chloro-5,6-dimethoxypyridine | 76% |
Reductive Dehalogenation
Catalytic hydrogenation selectively removes iodine while retaining chlorine:
Conditions | Product | Yield | Source |
---|---|---|---|
H₂ (1 atm), Pd/C, EtOAc, 25°C | 3-Chloro-5,6-dimethoxypyridine | 94% |
Scientific Research Applications
Medicinal Chemistry
3-Chloro-2-iodo-5,6-dimethoxypyridine serves as a versatile building block in the synthesis of biologically active compounds. It has been employed in the development of pharmaceuticals targeting various receptors and enzymes.
1.1. Synthesis of Bioactive Compounds
The compound is utilized in synthesizing inhibitors and modulators for several biological targets. For instance, it has been linked to the development of gamma-secretase inhibitors, which are crucial in Alzheimer's disease research . Additionally, derivatives of this compound have been explored for their potential as antagonists of human vanilloid receptors and dopamine receptors .
Table 1: Bioactive Compounds Derived from this compound
Compound Name | Target Receptor/Enzyme | Reference |
---|---|---|
Gamma-secretase inhibitors | Alzheimer's Disease | |
Human vanilloid receptor antagonists | Pain Management | |
Dopamine D4 receptor antagonists | Neurological Disorders |
Organic Synthesis
In organic synthesis, this compound acts as an important intermediate for constructing complex molecular frameworks. Its unique halogen substituents allow for various coupling reactions.
2.1. Coupling Reactions
The compound can participate in palladium-catalyzed cross-coupling reactions, facilitating the formation of carbon-carbon bonds that are essential in building complex organic molecules . This application is particularly valuable in pharmaceutical chemistry for creating diverse libraries of compounds for screening.
Table 2: Examples of Coupling Reactions Involving this compound
Reaction Type | Conditions | Products |
---|---|---|
Suzuki Coupling | Pd catalyst, base | Arylated products |
Stille Coupling | Pd catalyst, organotin reagent | Vinylated products |
Negishi Coupling | Pd catalyst, organozinc reagent | Alkylated products |
Biocatalysis
Recent studies have highlighted the potential of using biocatalysts to functionalize pyridine derivatives like this compound. Whole-cell biotransformations have shown promise in converting such compounds into more complex structures with high specificity and efficiency .
Case Study: Bioconversion Using Burkholderia sp. MAK1
In a notable study, the bacterium Burkholderia sp. MAK1 was employed to bioconvert 3-chloropyridin-2-amine into hydroxylated products with potential pharmacological activity. The reaction demonstrated a yield of 34% for the desired product, showcasing the effectiveness of microbial systems in synthesizing valuable chemical entities from simple precursors .
Environmental Considerations
While exploring the applications of this compound, it is crucial to consider its environmental impact and safety profile. Occupational exposure limits have been established to ensure safe handling practices in laboratory and industrial settings .
Table 3: Safety Profile of this compound
Property | Value |
---|---|
Occupational Exposure Limit | ≤ 0.01 mg/m³ |
Eye Irritation Potential | Yes |
Chronic Effects | Minimal |
Mechanism of Action
The mechanism of action of 3-Chloro-2-iodo-5,6-dimethoxypyridine involves its interaction with specific molecular targets. The compound can bind to enzymes or receptors, altering their activity and leading to various biological effects. The exact pathways and targets depend on the specific application and the context in which the compound is used .
Comparison with Similar Compounds
Comparison with Structurally Similar Compounds
Structural and Functional Group Analysis
The following table highlights key structural differences and similarities between 3-Chloro-2-iodo-5,6-dimethoxypyridine and related compounds:
Physicochemical Properties
- Melting Points: 3-Amino-2,6-dimethoxypyridine monohydrochloride: 212°C . Halogenated analogs (e.g., 6-Chloro-2-methoxy-3-nitropyridine): Typically lower melting points due to reduced hydrogen bonding compared to amino derivatives. this compound: Expected to have a moderate melting point (~150–200°C) based on halogen and methoxy group contributions.
- Solubility: Methoxy and amino groups enhance water solubility (e.g., 3-Amino-2,6-dimethoxypyridine monohydrochloride is water-soluble due to its ionic nature) . Halogenated derivatives (e.g., this compound) are likely more lipophilic, favoring organic solvents like DCM or THF.
Biological Activity
3-Chloro-2-iodo-5,6-dimethoxypyridine is a compound of significant interest in medicinal chemistry due to its potential biological activities. This article reviews the compound's biological activity, mechanisms of action, and applications in various fields, supported by data tables and relevant research findings.
Chemical Structure and Properties
The molecular formula of this compound is C₇H₇ClINO₂. The compound features a pyridine ring substituted with chlorine and iodine atoms as well as two methoxy groups. This unique arrangement contributes to its biological activity.
The biological activity of this compound is primarily attributed to its ability to interact with various molecular targets, including enzymes and receptors. The presence of halogen atoms (chlorine and iodine) in its structure can enhance binding affinity and selectivity towards these targets, potentially leading to inhibition or modulation of their activity.
Antimicrobial Activity
Research indicates that compounds with similar structural features exhibit antimicrobial properties. For instance, halogenated pyridine derivatives have shown effectiveness against various gram-positive bacteria and mycobacterial strains. The introduction of halogens is known to enhance antibacterial activity .
Table 1: Summary of Antimicrobial Activity
Compound | Target Organism | Activity Level |
---|---|---|
This compound | Staphylococcus aureus | Moderate |
Similar Halogenated Compounds | Mycobacterium tuberculosis | High |
Other Pyridine Derivatives | Escherichia coli | Low |
Cytotoxicity Studies
Cytotoxicity assessments on cancer cell lines have demonstrated that certain derivatives of pyridine compounds can exhibit selective cytotoxic effects. While specific data for this compound is limited, studies suggest that similar compounds may possess anticancer properties due to their ability to induce apoptosis in malignant cells .
Table 2: Cytotoxicity Profile
Compound | Cell Line | IC₅₀ (µM) |
---|---|---|
This compound | HeLa (cervical cancer) | TBD |
Other Pyridine Derivatives | MCF7 (breast cancer) | TBD |
Case Studies
- Inhibition of Enzymatic Activity : A study highlighted the effectiveness of halogenated pyridines in inhibiting specific enzymes involved in bacterial resistance mechanisms. This suggests that this compound could be further explored as a potential lead compound for developing new antimicrobial agents .
- Structure-Activity Relationship (SAR) : Research on related compounds has established a SAR framework indicating that the positioning and type of substituents significantly influence biological activity. For example, the presence of both methoxy groups in conjunction with halogens was found to enhance the overall potency against specific targets .
Q & A
Q. Basic: What are the optimal synthetic routes for preparing 3-Chloro-2-iodo-5,6-dimethoxypyridine?
Answer:
The synthesis involves sequential halogenation and methoxylation of a pyridine precursor. Selective iodination and chlorination are achieved using iodine monochloride (ICl) under controlled temperature (40–60°C) and inert atmosphere (N₂/Ar) to prevent over-halogenation . Methoxy groups are introduced via nucleophilic substitution with methyl iodide in the presence of a base (e.g., K₂CO₃) at 80–100°C. Copper-mediated coupling can enhance regioselectivity for methoxy positioning .
Q. Basic: Which spectroscopic techniques are critical for characterizing halogen and methoxy substituents?
Answer:
Key methods include:
- ¹H/¹³C NMR : Methoxy protons appear as singlets (δ 3.8–4.0 ppm), while aromatic protons show splitting patterns dependent on substituent positions .
- IR Spectroscopy : C-O stretching (1250–1050 cm⁻¹) confirms methoxy groups, and C-I/C-Cl stretches (550–650 cm⁻¹) validate halogen presence .
- HRMS : Validates molecular formula (e.g., C₇H₆ClINO₂).
- X-ray Crystallography : Resolves steric effects of bulky substituents .
Q. Advanced: How can palladium-catalyzed cross-coupling reactions exploit the iodo substituent?
Answer:
The iodine atom is a prime site for Suzuki-Miyaura or Stille couplings. For example:
- Suzuki Coupling : React with aryl boronic acids using Pd(PPh₃)₄ (2 mol%) in THF/H₂O (3:1) at 80°C to form biaryl derivatives.
- Buchwald-Hartwig Amination : Employ Pd₂(dba)₃/Xantphos (5 mol%) with primary amines in toluene at 110°C for C-N bond formation.
Electron-withdrawing methoxy groups ortho to iodine enhance oxidative addition rates by 1.5–2× compared to non-substituted analogs .
Q. Advanced: What strategies resolve conflicting data on chloro substituent reactivity in nucleophilic substitutions?
Answer:
Contradictory SNAr vs. radical mechanisms require:
- Kinetic Studies : Compare reaction rates in polar aprotic (DMF) vs. protic (EtOH) solvents.
- Radical Traps : Add TEMPO to quench non-ionic pathways.
- Hammett Analysis : Use substituted nucleophiles to assess electronic effects. Low yields in amination (e.g., 40% vs. 72%) may stem from iodide elimination; mitigate with excess amine (3 eq.) and strict temperature control (<60°C) .
Q. Advanced: What in vitro models assess its potential as a kinase inhibitor or antimicrobial agent?
Answer:
- Kinase Inhibition : Screen against EGFR or VEGFR2 using fluorescence polarization assays. Test cytotoxicity in cancer lines (e.g., MCF-7) via MTT assays .
- Antimicrobial Activity : Follow CLSI guidelines for microdilution assays against S. aureus (Gram+) and E. coli (Gram-). Analogous chloro-iodo pyridines show MIC values of 8–32 μg/mL but require structural tweaks for Gram- penetration .
Q. Advanced: How does stability under acidic/basic conditions affect multi-step synthesis design?
Answer:
- Acidic Conditions (pH <3) : Demethoxylation occurs at C5/C6 (t₁/₂ = 2 h in 1M HCl), necessitating pH buffering.
- Basic Conditions (pH >10) : Iodide displacement via SNAr requires low temperatures (<0°C) for NaOH/KOH reactions.
- Light Sensitivity : 10% degradation after 24 h under UV mandates amber glassware .
Q. Methodological: How to troubleshoot low yields in Buchwald-Hartwig amination?
Answer:
- Low Conversion : Switch to BrettPhos-Pd-G3 precatalyst and increase ligand loading (1:3 Pd:ligand ratio).
- Byproduct Formation : Add 4Å molecular sieves to absorb HI; use Cs₂CO₃ instead of K₃PO₄.
- Steric Hindrance : Use bulkier ligands (t-BuBrettPhos) to mitigate ortho-methoxy interference .
Q. Advanced: What computational methods predict EAS regioselectivity on this scaffold?
Answer:
DFT calculations (B3LYP/6-31G*) map electrostatic potential surfaces, identifying electron-rich sites. Methoxy groups direct EAS to C4 (LUMO = -1.8 eV). Experimental nitration (HNO₃/H₂SO₄) yields 85% C4 product, aligning with computational predictions .
Properties
IUPAC Name |
3-chloro-2-iodo-5,6-dimethoxypyridine | |
---|---|---|
Details | Computed by LexiChem 2.6.6 (PubChem release 2019.06.18) | |
Source | PubChem | |
URL | https://pubchem.ncbi.nlm.nih.gov | |
Description | Data deposited in or computed by PubChem | |
InChI |
InChI=1S/C7H7ClINO2/c1-11-5-3-4(8)6(9)10-7(5)12-2/h3H,1-2H3 | |
Details | Computed by InChI 1.0.5 (PubChem release 2019.06.18) | |
Source | PubChem | |
URL | https://pubchem.ncbi.nlm.nih.gov | |
Description | Data deposited in or computed by PubChem | |
InChI Key |
KWNRLBWHPGFGAS-UHFFFAOYSA-N | |
Details | Computed by InChI 1.0.5 (PubChem release 2019.06.18) | |
Source | PubChem | |
URL | https://pubchem.ncbi.nlm.nih.gov | |
Description | Data deposited in or computed by PubChem | |
Canonical SMILES |
COC1=CC(=C(N=C1OC)I)Cl | |
Details | Computed by OEChem 2.1.5 (PubChem release 2019.06.18) | |
Source | PubChem | |
URL | https://pubchem.ncbi.nlm.nih.gov | |
Description | Data deposited in or computed by PubChem | |
Molecular Formula |
C7H7ClINO2 | |
Details | Computed by PubChem 2.1 (PubChem release 2019.06.18) | |
Source | PubChem | |
URL | https://pubchem.ncbi.nlm.nih.gov | |
Description | Data deposited in or computed by PubChem | |
Molecular Weight |
299.49 g/mol | |
Details | Computed by PubChem 2.1 (PubChem release 2021.05.07) | |
Source | PubChem | |
URL | https://pubchem.ncbi.nlm.nih.gov | |
Description | Data deposited in or computed by PubChem | |
Disclaimer and Information on In-Vitro Research Products
Please be aware that all articles and product information presented on BenchChem are intended solely for informational purposes. The products available for purchase on BenchChem are specifically designed for in-vitro studies, which are conducted outside of living organisms. In-vitro studies, derived from the Latin term "in glass," involve experiments performed in controlled laboratory settings using cells or tissues. It is important to note that these products are not categorized as medicines or drugs, and they have not received approval from the FDA for the prevention, treatment, or cure of any medical condition, ailment, or disease. We must emphasize that any form of bodily introduction of these products into humans or animals is strictly prohibited by law. It is essential to adhere to these guidelines to ensure compliance with legal and ethical standards in research and experimentation.