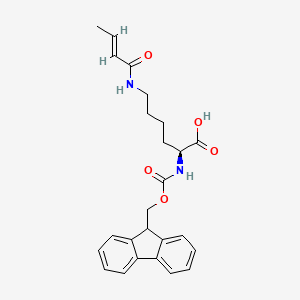
Fmoc-Lys(Crotonyl)-OH
Overview
Description
Fmoc-Lys(Crotonyl)-OH, also known as 9-fluorenylmethyloxycarbonyl-L-lysine (crotonyl), is a derivative of lysine, an essential amino acid. The Fmoc group is commonly used in peptide synthesis as a protecting group for the amino group, while the crotonyl group is a type of acyl group derived from crotonic acid. This compound is particularly useful in the field of peptide synthesis and modification due to its unique chemical properties.
Mechanism of Action
Target of Action
Fmoc-Lys(Crotonyl)-OH is a modified amino acid that primarily targets the formation of functional materials, such as hydrogels . These hydrogels are often used in biomedical applications, including drug delivery and diagnostic tools for imaging .
Mode of Action
The compound interacts with its targets through a process known as self-assembly . This process is driven by the inherent hydrophobicity and aromaticity of the Fmoc moiety, which promotes the association of building blocks . The self-assembly of Fmoc-modified amino acids and short peptides occurs in a kinetically rapid and thermodynamically rigid manner .
Biochemical Pathways
The biochemical pathways affected by this compound primarily involve the self-assembly of functional molecules. This includes Fmoc-modified individual amino acids, di- and tripeptides, and tetra- and pentapeptides . The self-assembly process is influenced by various non-covalent interactions, including hydrophobic interactions from the fluorenyl ring and steric optimization from the linker .
Pharmacokinetics
It’s known that the compound can form hydrogels, which have potential applications in drug delivery . The formation of these hydrogels could influence the bioavailability of drugs delivered using this compound.
Result of Action
The molecular and cellular effects of this compound’s action primarily involve the formation of hydrogels . These hydrogels can support cell adhesion, survival, and duplication . Additionally, the compound can induce gel formation, which is crucial for its applications in biomedical fields .
Action Environment
The action of this compound can be influenced by various environmental factors. For instance, the pH and buffer ions can affect the self-assembly of the compound to gel formation . Moreover, physical and thermal stimuli can be used for solubilizing this compound above the critical concentration to induce gel formation .
Biochemical Analysis
Biochemical Properties
Fmoc-Lys(Crotonyl)-OH is involved in various biochemical reactions. It interacts with enzymes, proteins, and other biomolecules. The Fmoc group promotes the association of building blocks, which is crucial for the self-organization of functional molecules .
Molecular Mechanism
The molecular mechanism of this compound involves its effects at the molecular level. It exerts its effects through binding interactions with biomolecules, potential enzyme inhibition or activation, and changes in gene expression
Preparation Methods
Synthetic Routes and Reaction Conditions
The synthesis of Fmoc-Lys(Crotonyl)-OH typically involves the protection of the lysine amino group with the Fmoc group, followed by the introduction of the crotonyl group. The process generally starts with the reaction of lysine with 9-fluorenylmethyloxycarbonyl chloride in the presence of a base such as triethylamine to form Fmoc-Lys. The crotonyl group is then introduced by reacting Fmoc-Lys with crotonic anhydride or crotonyl chloride under basic conditions .
Industrial Production Methods
Industrial production of this compound follows similar synthetic routes but on a larger scale. The process involves the use of automated peptide synthesizers that can handle large quantities of reagents and solvents. The use of solid-phase peptide synthesis (SPPS) techniques is common, where the peptide chain is assembled on a solid support, allowing for efficient separation and purification of the product .
Chemical Reactions Analysis
Types of Reactions
Fmoc-Lys(Crotonyl)-OH can undergo various chemical reactions, including:
Oxidation: The crotonyl group can be oxidized to form corresponding carboxylic acids.
Reduction: The crotonyl group can be reduced to form saturated derivatives.
Substitution: The Fmoc group can be removed under basic conditions to expose the amino group for further reactions.
Common Reagents and Conditions
Oxidation: Common oxidizing agents include potassium permanganate and chromium trioxide.
Reduction: Reducing agents such as lithium aluminum hydride or sodium borohydride are used.
Substitution: The Fmoc group is typically removed using piperidine in dimethylformamide.
Major Products Formed
Oxidation: Formation of carboxylic acids.
Reduction: Formation of saturated lysine derivatives.
Substitution: Exposure of the free amino group for further peptide coupling reactions.
Scientific Research Applications
Role in Post-Translational Modifications
Crotonylation, the modification of lysine residues with crotonyl groups, is a significant post-translational modification that influences protein function and interaction. Fmoc-Lys(Crotonyl)-OH serves as a key building block for synthesizing peptides that mimic crotonylated proteins. This allows researchers to investigate the biological implications of crotonylation in various cellular processes.
Case Study: Sirtuin Enzymes and Decrotonylation
A notable application of this compound is in studying the decrotonylation activity of sirtuin enzymes (Sirt1, Sirt2, and Sirt3). These enzymes have been shown to hydrolyze crotonylated peptides, revealing insights into their catalytic mechanisms and substrate specificity. For instance, research demonstrated that Sirt3 effectively decrotonylates histone peptides, highlighting the importance of specific interactions between the enzyme and the crotonyl group .
The study utilized liquid chromatography-mass spectrometry to monitor the hydrolysis of crotonylated peptides, providing quantitative data on enzyme kinetics. The findings indicated that the decrotonylation process is NAD-dependent and involves complex binding interactions between the enzyme and substrate .
Synthetic Biology Applications
This compound is also utilized in synthetic biology for constructing modified peptides and proteins. Its incorporation into peptide sequences allows for the study of how modifications affect protein stability, folding, and interactions.
Example: Fluorogenic Probes
Recent advancements have seen this compound being used to create fluorogenic probes that detect lysine deacetylase activity. These probes leverage the unique properties of crotonylated lysine to enable real-time monitoring of enzymatic reactions. The N-decrotonylase activity of histone deacetylase 3 (HDAC3) was successfully detected using a chemical probe containing an N-crotonyl-lysine group, demonstrating its utility in enzymatic assays .
Implications in Metabolism and Disease
The study of this compound extends to understanding metabolic regulation and its implications in diseases such as cancer. Crotonylation has been linked to metabolic pathways and gene expression regulation. By employing this compound in experimental designs, researchers can elucidate how metabolic states influence protein modifications and subsequent cellular outcomes.
Data Table: Summary of Applications
Application Area | Description | Key Findings/Implications |
---|---|---|
Post-Translational Modifications | Used as a building block for synthesizing crotonylated peptides | Critical for studying protein interactions and functions |
Enzymatic Studies | Investigating decrotonylation by sirtuins | Reveals catalytic mechanisms; NAD-dependence identified |
Synthetic Biology | Creating modified peptides for stability and interaction studies | Development of fluorogenic probes for real-time enzymatic assays |
Metabolic Regulation | Understanding links between crotonylation and metabolic pathways | Insights into disease mechanisms, particularly in cancer |
Comparison with Similar Compounds
Similar Compounds
Fmoc-Lys(Boc)-OH: Another lysine derivative with a tert-butyloxycarbonyl protecting group.
Fmoc-Lys(Ac)-OH: A lysine derivative with an acetyl protecting group.
Fmoc-Lys(Mtt)-OH: A lysine derivative with a 4-methyltrityl protecting group.
Uniqueness
This makes it particularly useful in the study of post-translational modifications and the development of peptide-based therapeutics .
Biological Activity
Fmoc-Lys(Crotonyl)-OH is a derivative of the amino acid L-lysine, characterized by the presence of a fluorenylmethyloxycarbonyl (Fmoc) protecting group at the N-terminus and a crotonyl group attached to the side chain. This compound plays a significant role in peptide synthesis and has garnered interest due to its biological activities, particularly in the context of lysine acylation.
Structure and Properties
- Chemical Name : N-alpha-(9-Fluorenylmethyloxycarbonyl)-N-epsilon-crotonyl-L-lysine
- Molecular Formula : C₁₄H₁₉N₃O₃
- Molecular Weight : 273.32 g/mol
The Fmoc group allows for selective deprotection during solid-phase peptide synthesis (SPPS), facilitating the incorporation of lysine into peptide sequences. The crotonyl modification is particularly noteworthy as it is associated with various biological functions, including histone modification.
Role in Histone Modification
Recent studies have highlighted the significance of lysine acylations, including crotonylation, in regulating gene expression through epigenetic mechanisms. Crotonylation has been shown to recruit specific bromodomains, which are protein motifs that recognize acetylated lysines on histones, thereby influencing chromatin structure and function .
Key Findings
- Bromodomain Interactions : Research indicates that several bromodomains (e.g., BRD7, BRD9) specifically bind to crotonylated lysines. This interaction plays a crucial role in modulating transcriptional activity .
- Metabolic Pathways : Crotonyl-CoA, a metabolite involved in fatty acid metabolism, has been linked to the formation of crotonylated lysines on histones, suggesting a metabolic regulation of gene expression .
Synthesis and Application in Research
This compound serves as a versatile building block in peptide synthesis. Its ability to undergo acylation reactions allows researchers to create peptides that mimic natural proteins or modify existing ones for functional studies.
Applications in Peptide Synthesis
- Solid-Phase Peptide Synthesis (SPPS) : The compound is utilized as a precursor in SPPS, enabling the creation of peptides with specific post-translational modifications.
- Investigating Protein Function : By incorporating this compound into peptides, researchers can study the effects of crotonylation on protein interactions and functions.
Case Studies
- Histone Acetylation and Crotonylation : A study demonstrated that crotonylation is not merely an alternative to acetylation but serves distinct functions in transcriptional regulation. The use of synthetic peptides containing this compound allowed for detailed analysis of these modifications' effects on chromatin dynamics .
- Protein Interaction Studies : In another investigation, peptides synthesized with this compound were used to assess binding affinities with bromodomains, revealing insights into how crotonylation influences protein-protein interactions within the nucleus .
Comparative Analysis of Lysine Modifications
The following table summarizes key differences between various lysine modifications relevant to biological activity:
Modification Type | Biological Function | Key Enzymes Involved |
---|---|---|
Acetylation | Gene activation; chromatin relaxation | Histone acetyltransferases (HATs) |
Crotonylation | Gene regulation; recruitment of bromodomains | Enzymes involved in fatty acid metabolism |
Succinylation | Metabolic regulation; affects protein stability | Succinyl-CoA synthetase |
Properties
IUPAC Name |
(2S)-6-[[(E)-but-2-enoyl]amino]-2-(9H-fluoren-9-ylmethoxycarbonylamino)hexanoic acid | |
---|---|---|
Details | Computed by Lexichem TK 2.7.0 (PubChem release 2021.05.07) | |
Source | PubChem | |
URL | https://pubchem.ncbi.nlm.nih.gov | |
Description | Data deposited in or computed by PubChem | |
InChI |
InChI=1S/C25H28N2O5/c1-2-9-23(28)26-15-8-7-14-22(24(29)30)27-25(31)32-16-21-19-12-5-3-10-17(19)18-11-4-6-13-20(18)21/h2-6,9-13,21-22H,7-8,14-16H2,1H3,(H,26,28)(H,27,31)(H,29,30)/b9-2+/t22-/m0/s1 | |
Details | Computed by InChI 1.0.6 (PubChem release 2021.05.07) | |
Source | PubChem | |
URL | https://pubchem.ncbi.nlm.nih.gov | |
Description | Data deposited in or computed by PubChem | |
InChI Key |
WFNXIABNUWPOIE-INZNARQDSA-N | |
Details | Computed by InChI 1.0.6 (PubChem release 2021.05.07) | |
Source | PubChem | |
URL | https://pubchem.ncbi.nlm.nih.gov | |
Description | Data deposited in or computed by PubChem | |
Canonical SMILES |
CC=CC(=O)NCCCCC(C(=O)O)NC(=O)OCC1C2=CC=CC=C2C3=CC=CC=C13 | |
Details | Computed by OEChem 2.3.0 (PubChem release 2021.05.07) | |
Source | PubChem | |
URL | https://pubchem.ncbi.nlm.nih.gov | |
Description | Data deposited in or computed by PubChem | |
Isomeric SMILES |
C/C=C/C(=O)NCCCC[C@@H](C(=O)O)NC(=O)OCC1C2=CC=CC=C2C3=CC=CC=C13 | |
Details | Computed by OEChem 2.3.0 (PubChem release 2021.05.07) | |
Source | PubChem | |
URL | https://pubchem.ncbi.nlm.nih.gov | |
Description | Data deposited in or computed by PubChem | |
Molecular Formula |
C25H28N2O5 | |
Details | Computed by PubChem 2.1 (PubChem release 2021.05.07) | |
Source | PubChem | |
URL | https://pubchem.ncbi.nlm.nih.gov | |
Description | Data deposited in or computed by PubChem | |
Molecular Weight |
436.5 g/mol | |
Details | Computed by PubChem 2.1 (PubChem release 2021.05.07) | |
Source | PubChem | |
URL | https://pubchem.ncbi.nlm.nih.gov | |
Description | Data deposited in or computed by PubChem | |
Disclaimer and Information on In-Vitro Research Products
Please be aware that all articles and product information presented on BenchChem are intended solely for informational purposes. The products available for purchase on BenchChem are specifically designed for in-vitro studies, which are conducted outside of living organisms. In-vitro studies, derived from the Latin term "in glass," involve experiments performed in controlled laboratory settings using cells or tissues. It is important to note that these products are not categorized as medicines or drugs, and they have not received approval from the FDA for the prevention, treatment, or cure of any medical condition, ailment, or disease. We must emphasize that any form of bodily introduction of these products into humans or animals is strictly prohibited by law. It is essential to adhere to these guidelines to ensure compliance with legal and ethical standards in research and experimentation.