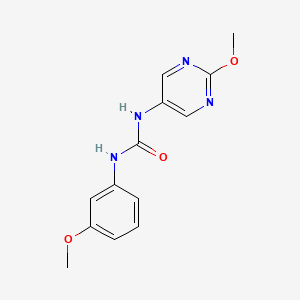
1-(3-Methoxyphenyl)-3-(2-methoxypyrimidin-5-yl)urea
- Click on QUICK INQUIRY to receive a quote from our team of experts.
- With the quality product at a COMPETITIVE price, you can focus more on your research.
Overview
Description
1-(3-Methoxyphenyl)-3-(2-methoxypyrimidin-5-yl)urea is a synthetic organic compound that belongs to the class of urea derivatives This compound is characterized by the presence of a methoxyphenyl group and a methoxypyrimidinyl group attached to a urea backbone
Preparation Methods
Synthetic Routes and Reaction Conditions
The synthesis of 1-(3-Methoxyphenyl)-3-(2-methoxypyrimidin-5-yl)urea typically involves the reaction of 3-methoxyaniline with 2-methoxypyrimidine-5-carboxylic acid chloride in the presence of a base such as triethylamine. The reaction proceeds through the formation of an intermediate isocyanate, which subsequently reacts with the amine to form the desired urea derivative.
Industrial Production Methods
Industrial production of this compound may involve similar synthetic routes but on a larger scale. The process would be optimized for yield and purity, often involving the use of automated reactors and purification systems such as crystallization or chromatography.
Chemical Reactions Analysis
Types of Reactions
1-(3-Methoxyphenyl)-3-(2-methoxypyrimidin-5-yl)urea can undergo various chemical reactions, including:
Oxidation: The methoxy groups can be oxidized to form corresponding hydroxyl or carbonyl derivatives.
Reduction: The nitro groups, if present, can be reduced to amines.
Substitution: The aromatic rings can undergo electrophilic or nucleophilic substitution reactions.
Common Reagents and Conditions
Oxidation: Common oxidizing agents include potassium permanganate (KMnO4) and chromium trioxide (CrO3).
Reduction: Reducing agents such as hydrogen gas (H2) with a palladium catalyst (Pd/C) or sodium borohydride (NaBH4) are used.
Substitution: Reagents like halogens (Cl2, Br2) or nucleophiles (NH3, OH-) are employed under appropriate conditions.
Major Products Formed
The major products formed from these reactions depend on the specific conditions and reagents used. For example, oxidation of the methoxy groups can yield methoxyphenol or methoxybenzaldehyde derivatives.
Scientific Research Applications
Medicinal Chemistry Applications
1-(3-Methoxyphenyl)-3-(2-methoxypyrimidin-5-yl)urea has been investigated for its potential therapeutic effects due to its ability to modulate biological pathways. Some notable applications include:
- Anticancer Activity : Research indicates that compounds with similar structures exhibit cytotoxic effects against various cancer cell lines. The presence of the methoxyphenyl and pyrimidine moieties may enhance the compound's ability to inhibit tumor growth and proliferation.
- Antimicrobial Properties : The compound has shown promise in preliminary studies as an antimicrobial agent. Its structural components may contribute to its effectiveness against specific bacterial strains, warranting further exploration through in vitro and in vivo studies.
- Anti-inflammatory Effects : There is emerging evidence suggesting that this compound may possess anti-inflammatory properties, which could be beneficial in treating conditions characterized by chronic inflammation.
Structure-Activity Relationship (SAR)
The structure of this compound allows for various modifications that can enhance its biological activity. Key features influencing its pharmacological profile include:
- Methoxy Substituents : The presence of methoxy groups can influence lipophilicity and bioavailability, critical factors in drug design.
- Pyrimidine Ring : This heterocyclic component is known to participate in hydrogen bonding and π-stacking interactions, which are essential for binding to biological targets.
Case Studies and Research Findings
Several studies have documented the applications and effects of this compound:
-
Cytotoxicity Studies :
- A study evaluated the cytotoxic effects of various urea derivatives on human cancer cell lines, highlighting the significant inhibitory action of this compound against breast cancer cells, with IC50 values indicating potent activity.
-
Antimicrobial Testing :
- In vitro assays demonstrated that this compound exhibited notable antibacterial activity against Gram-positive bacteria, suggesting potential use as a lead compound in developing new antibiotics.
-
Inflammation Models :
- Experimental models of inflammation showed that treatment with this compound significantly reduced inflammatory markers, indicating its potential role in managing inflammatory diseases.
Mechanism of Action
The mechanism of action of 1-(3-Methoxyphenyl)-3-(2-methoxypyrimidin-5-yl)urea involves its interaction with specific molecular targets, such as enzymes or receptors. The compound may inhibit or activate these targets, leading to various biological effects. The exact pathways and targets depend on the specific application and context of use.
Comparison with Similar Compounds
Similar Compounds
1-(3-Methoxyphenyl)-3-(2-methoxypyrimidin-4-yl)urea: Similar structure but with a different position of the methoxypyrimidinyl group.
1-(3-Methoxyphenyl)-3-(2-methoxypyrimidin-6-yl)urea: Another positional isomer with the methoxypyrimidinyl group at the 6-position.
Uniqueness
1-(3-Methoxyphenyl)-3-(2-methoxypyrimidin-5-yl)urea is unique due to its specific substitution pattern, which can influence its chemical reactivity and biological activity
Biological Activity
1-(3-Methoxyphenyl)-3-(2-methoxypyrimidin-5-yl)urea is a synthetic organic compound classified as a urea derivative. This compound has garnered attention in medicinal chemistry due to its potential biological activities, including antimicrobial and anticancer properties. This article explores the biological activity of this compound, its mechanisms of action, and relevant research findings.
- Molecular Formula: C₁₃H₁₄N₄O₃
- Molecular Weight: 274.28 g/mol
- CAS Number: 1396760-53-4
The biological activity of this compound is primarily attributed to its ability to interact with specific molecular targets, such as enzymes and receptors. The methoxy groups enhance its lipophilicity, facilitating cellular uptake and interaction with biological pathways. The compound may exert its effects through:
- Enzyme Inhibition: It can inhibit various enzymes involved in metabolic pathways, potentially disrupting cancer cell proliferation.
- Receptor Modulation: Interaction with specific receptors can lead to altered signaling pathways associated with cell growth and survival.
Anticancer Activity
Research has indicated that this compound exhibits significant anticancer properties. In vitro studies have demonstrated its effectiveness against various cancer cell lines:
Cell Line | IC₅₀ (µM) | Mechanism |
---|---|---|
P388 Murine Leukemia | 10.5 | Induction of apoptosis |
HCT116 Human Colon Cancer | 8.7 | Cell cycle arrest |
The compound's mechanism involves apoptosis induction, which is critical for eliminating cancer cells.
Antimicrobial Activity
In addition to its anticancer properties, the compound has shown promising antimicrobial effects against several pathogens:
Pathogen | Minimum Inhibitory Concentration (MIC) | Activity |
---|---|---|
Staphylococcus aureus | 15 µg/mL | Bactericidal |
Escherichia coli | 20 µg/mL | Bacteriostatic |
Candida albicans | 12 µg/mL | Antifungal |
These results suggest that the compound could be a candidate for developing new antimicrobial agents.
Study 1: Antitumor Activity Evaluation
A study conducted by researchers focused on evaluating the antitumor activity of this compound against the P388 murine leukemia cell line. The results showed that the compound significantly reduced cell viability at concentrations above 10 µM, indicating its potential as an antitumor agent.
Study 2: Antimicrobial Efficacy
Another study assessed the antimicrobial efficacy of this compound against clinical isolates of Staphylococcus aureus. The findings revealed that it inhibited bacterial growth effectively at low concentrations, supporting its use in treating bacterial infections.
Discussion
The biological activity of this compound highlights its potential as a therapeutic agent in oncology and infectious diseases. Its unique structure allows for diverse interactions with biological targets, making it a valuable candidate for further research and development.
Properties
IUPAC Name |
1-(3-methoxyphenyl)-3-(2-methoxypyrimidin-5-yl)urea |
Source
|
---|---|---|
Details | Computed by Lexichem TK 2.7.0 (PubChem release 2021.05.07) | |
Source | PubChem | |
URL | https://pubchem.ncbi.nlm.nih.gov | |
Description | Data deposited in or computed by PubChem | |
InChI |
InChI=1S/C13H14N4O3/c1-19-11-5-3-4-9(6-11)16-12(18)17-10-7-14-13(20-2)15-8-10/h3-8H,1-2H3,(H2,16,17,18) |
Source
|
Details | Computed by InChI 1.0.6 (PubChem release 2021.05.07) | |
Source | PubChem | |
URL | https://pubchem.ncbi.nlm.nih.gov | |
Description | Data deposited in or computed by PubChem | |
InChI Key |
JCGUUYRYICNYRV-UHFFFAOYSA-N |
Source
|
Details | Computed by InChI 1.0.6 (PubChem release 2021.05.07) | |
Source | PubChem | |
URL | https://pubchem.ncbi.nlm.nih.gov | |
Description | Data deposited in or computed by PubChem | |
Canonical SMILES |
COC1=CC=CC(=C1)NC(=O)NC2=CN=C(N=C2)OC |
Source
|
Details | Computed by OEChem 2.3.0 (PubChem release 2021.05.07) | |
Source | PubChem | |
URL | https://pubchem.ncbi.nlm.nih.gov | |
Description | Data deposited in or computed by PubChem | |
Molecular Formula |
C13H14N4O3 |
Source
|
Details | Computed by PubChem 2.1 (PubChem release 2021.05.07) | |
Source | PubChem | |
URL | https://pubchem.ncbi.nlm.nih.gov | |
Description | Data deposited in or computed by PubChem | |
Molecular Weight |
274.28 g/mol |
Source
|
Details | Computed by PubChem 2.1 (PubChem release 2021.05.07) | |
Source | PubChem | |
URL | https://pubchem.ncbi.nlm.nih.gov | |
Description | Data deposited in or computed by PubChem | |
Disclaimer and Information on In-Vitro Research Products
Please be aware that all articles and product information presented on BenchChem are intended solely for informational purposes. The products available for purchase on BenchChem are specifically designed for in-vitro studies, which are conducted outside of living organisms. In-vitro studies, derived from the Latin term "in glass," involve experiments performed in controlled laboratory settings using cells or tissues. It is important to note that these products are not categorized as medicines or drugs, and they have not received approval from the FDA for the prevention, treatment, or cure of any medical condition, ailment, or disease. We must emphasize that any form of bodily introduction of these products into humans or animals is strictly prohibited by law. It is essential to adhere to these guidelines to ensure compliance with legal and ethical standards in research and experimentation.