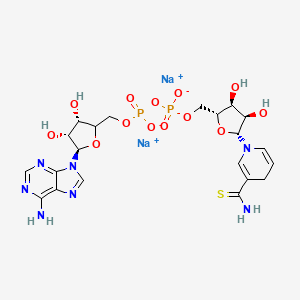
beta-Thionicotinamide adenine dinucleotide, reduced form disodium salt
- Click on QUICK INQUIRY to receive a quote from our team of experts.
- With the quality product at a COMPETITIVE price, you can focus more on your research.
Overview
Description
Beta-Thionicotinamide adenine dinucleotide, reduced form disodium salt: is a derivative of nicotinamide adenine dinucleotide, a coenzyme found in all living cells. This compound plays a crucial role in various biochemical processes, particularly in redox reactions where it acts as an electron donor. Its unique structure includes a thioamide group, which distinguishes it from other nicotinamide adenine dinucleotide derivatives.
Preparation Methods
Synthetic Routes and Reaction Conditions
The synthesis of beta-Thionicotinamide adenine dinucleotide, reduced form disodium salt typically involves the following steps:
Thioamide Formation:
Reduction: The compound is then reduced using reducing agents such as sodium borohydride or hydrogen gas in the presence of a catalyst.
Disodium Salt Formation: The final step involves the neutralization of the compound with sodium hydroxide to form the disodium salt.
Industrial Production Methods
Industrial production of this compound follows similar synthetic routes but on a larger scale. The process is optimized for higher yields and purity, often involving continuous flow reactors and advanced purification techniques such as high-performance liquid chromatography.
Chemical Reactions Analysis
Types of Reactions
Oxidation-Reduction Reactions: Beta-Thionicotinamide adenine dinucleotide, reduced form disodium salt primarily participates in redox reactions, where it donates electrons to various substrates.
Substitution Reactions: The thioamide group can undergo substitution reactions with nucleophiles, leading to the formation of various derivatives.
Common Reagents and Conditions
Oxidizing Agents: Common oxidizing agents include potassium permanganate and hydrogen peroxide.
Reducing Agents: Sodium borohydride and lithium aluminum hydride are frequently used.
Catalysts: Palladium on carbon and platinum are typical catalysts for hydrogenation reactions.
Major Products
The major products formed from these reactions depend on the specific conditions and reagents used. For instance, oxidation reactions typically yield oxidized forms of the compound, while substitution reactions can produce a variety of thioamide derivatives.
Scientific Research Applications
Chemistry
In chemistry, beta-Thionicotinamide adenine dinucleotide, reduced form disodium salt is used as a model compound to study redox reactions and electron transfer mechanisms. Its unique properties make it a valuable tool for investigating the behavior of thioamide-containing molecules.
Biology
In biological research, this compound is utilized to study cellular redox states and metabolic pathways. It serves as a probe to understand the role of nicotinamide adenine dinucleotide derivatives in cellular processes.
Medicine
This compound has potential therapeutic applications due to its ability to modulate redox states in cells. It is being investigated for its role in treating oxidative stress-related diseases and conditions.
Industry
In the industrial sector, this compound is used in the development of biosensors and diagnostic assays. Its redox properties are harnessed to create sensitive and specific detection systems for various analytes.
Mechanism of Action
The mechanism of action of beta-Thionicotinamide adenine dinucleotide, reduced form disodium salt involves its role as an electron donor in redox reactions. It interacts with various enzymes and proteins, facilitating the transfer of electrons and modulating the redox state of cells. The thioamide group enhances its reactivity, making it a potent redox agent.
Comparison with Similar Compounds
Similar Compounds
- Nicotinamide adenine dinucleotide (NAD)
- Nicotinamide adenine dinucleotide, reduced form (NADH)
- Thionicotinamide adenine dinucleotide
Uniqueness
Beta-Thionicotinamide adenine dinucleotide, reduced form disodium salt is unique due to the presence of the thioamide group, which imparts distinct chemical and biological properties. This modification enhances its reactivity in redox reactions and makes it a valuable tool for studying electron transfer processes.
By comparing it with similar compounds, it is evident that the thioamide group provides additional functionality, making it more versatile in various applications.
Properties
IUPAC Name |
disodium;[[(3S,4R,5R)-5-(6-aminopurin-9-yl)-3,4-dihydroxyoxolan-2-yl]methoxy-oxidophosphoryl] [(2R,3S,4R,5R)-5-(3-carbamothioyl-4H-pyridin-1-yl)-3,4-dihydroxyoxolan-2-yl]methyl phosphate |
Source
|
---|---|---|
Details | Computed by Lexichem TK 2.7.0 (PubChem release 2021.05.07) | |
Source | PubChem | |
URL | https://pubchem.ncbi.nlm.nih.gov | |
Description | Data deposited in or computed by PubChem | |
InChI |
InChI=1S/C21H29N7O13P2S.2Na/c22-17-12-19(25-7-24-17)28(8-26-12)21-16(32)14(30)11(40-21)6-38-43(35,36)41-42(33,34)37-5-10-13(29)15(31)20(39-10)27-3-1-2-9(4-27)18(23)44;;/h1,3-4,7-8,10-11,13-16,20-21,29-32H,2,5-6H2,(H2,23,44)(H,33,34)(H,35,36)(H2,22,24,25);;/q;2*+1/p-2/t10-,11?,13-,14-,15-,16-,20-,21-;;/m1../s1 |
Source
|
Details | Computed by InChI 1.0.6 (PubChem release 2021.05.07) | |
Source | PubChem | |
URL | https://pubchem.ncbi.nlm.nih.gov | |
Description | Data deposited in or computed by PubChem | |
InChI Key |
XLEVNBOZUZYSIR-CQQDLCLESA-L |
Source
|
Details | Computed by InChI 1.0.6 (PubChem release 2021.05.07) | |
Source | PubChem | |
URL | https://pubchem.ncbi.nlm.nih.gov | |
Description | Data deposited in or computed by PubChem | |
Canonical SMILES |
C1C=CN(C=C1C(=S)N)C2C(C(C(O2)COP(=O)([O-])OP(=O)([O-])OCC3C(C(C(O3)N4C=NC5=C(N=CN=C54)N)O)O)O)O.[Na+].[Na+] |
Source
|
Details | Computed by OEChem 2.3.0 (PubChem release 2021.05.07) | |
Source | PubChem | |
URL | https://pubchem.ncbi.nlm.nih.gov | |
Description | Data deposited in or computed by PubChem | |
Isomeric SMILES |
C1C=CN(C=C1C(=S)N)[C@H]2[C@@H]([C@@H]([C@H](O2)COP(=O)([O-])OP(=O)([O-])OCC3[C@H]([C@H]([C@@H](O3)N4C=NC5=C(N=CN=C54)N)O)O)O)O.[Na+].[Na+] |
Source
|
Details | Computed by OEChem 2.3.0 (PubChem release 2021.05.07) | |
Source | PubChem | |
URL | https://pubchem.ncbi.nlm.nih.gov | |
Description | Data deposited in or computed by PubChem | |
Molecular Formula |
C21H27N7Na2O13P2S |
Source
|
Details | Computed by PubChem 2.1 (PubChem release 2021.05.07) | |
Source | PubChem | |
URL | https://pubchem.ncbi.nlm.nih.gov | |
Description | Data deposited in or computed by PubChem | |
Molecular Weight |
725.5 g/mol |
Source
|
Details | Computed by PubChem 2.1 (PubChem release 2021.05.07) | |
Source | PubChem | |
URL | https://pubchem.ncbi.nlm.nih.gov | |
Description | Data deposited in or computed by PubChem | |
Disclaimer and Information on In-Vitro Research Products
Please be aware that all articles and product information presented on BenchChem are intended solely for informational purposes. The products available for purchase on BenchChem are specifically designed for in-vitro studies, which are conducted outside of living organisms. In-vitro studies, derived from the Latin term "in glass," involve experiments performed in controlled laboratory settings using cells or tissues. It is important to note that these products are not categorized as medicines or drugs, and they have not received approval from the FDA for the prevention, treatment, or cure of any medical condition, ailment, or disease. We must emphasize that any form of bodily introduction of these products into humans or animals is strictly prohibited by law. It is essential to adhere to these guidelines to ensure compliance with legal and ethical standards in research and experimentation.