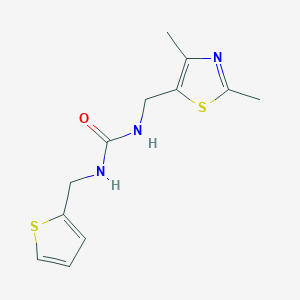
1-((2,4-Dimethylthiazol-5-yl)methyl)-3-(thiophen-2-ylmethyl)urea
Overview
Description
Compound A features a urea core linked to a 2,4-dimethylthiazole moiety and an indenopyrazole scaffold substituted with a methylpiperazine group.
Preparation Methods
Synthetic Routes and Reaction Conditions: The synthesis of 1-((2,4-Dimethylthiazol-5-yl)methyl)-3-(thiophen-2-ylmethyl)urea typically involves the following steps:
Formation of the Thiazole Ring: The thiazole ring can be synthesized through the Hantzsch thiazole synthesis, which involves the condensation of α-haloketones with thioamides.
Formation of the Thiophene Ring: The thiophene ring can be synthesized via the Gewald reaction, which involves the reaction of α-cyanoesters with elemental sulfur and ketones.
Coupling Reactions: The thiazole and thiophene rings are then coupled through a series of nucleophilic substitution reactions, often using reagents such as sodium hydride (NaH) or potassium carbonate (K2CO3) in polar aprotic solvents like dimethylformamide (DMF).
Urea Formation: The final step involves the formation of the urea linkage by reacting the intermediate with an isocyanate or by using phosgene derivatives under controlled conditions.
Industrial Production Methods: Industrial production of this compound would likely involve scaling up the laboratory synthesis methods, optimizing reaction conditions for higher yields and purity, and ensuring compliance with safety and environmental regulations. Continuous flow reactors and automated synthesis platforms may be employed to enhance efficiency and reproducibility.
Types of Reactions:
Oxidation: The compound can undergo oxidation reactions, particularly at the sulfur atoms in the thiazole and thiophene rings, using oxidizing agents such as hydrogen peroxide (H2O2) or m-chloroperbenzoic acid (m-CPBA).
Reduction: Reduction reactions can target the urea linkage or the aromatic rings, using reducing agents like lithium aluminum hydride (LiAlH4) or sodium borohydride (NaBH4).
Substitution: Electrophilic and nucleophilic substitution reactions can occur at the methyl groups or the aromatic rings, using reagents such as halogens (e.g., bromine) or nucleophiles (e.g., amines).
Common Reagents and Conditions:
Oxidation: H2O2, m-CPBA, acetic acid (AcOH) as a solvent.
Reduction: LiAlH4, NaBH4, tetrahydrofuran (THF) as a solvent.
Substitution: Halogens, amines, polar aprotic solvents like DMF or dimethyl sulfoxide (DMSO).
Major Products:
Oxidation: Sulfoxides or sulfones.
Reduction: Amines or alcohols.
Substitution: Halogenated or aminated derivatives.
Chemistry:
Catalysis: The compound can be used as a ligand in coordination chemistry, forming complexes with transition metals that act as catalysts in various organic reactions.
Materials Science: It can be incorporated into polymers or used as a building block for the synthesis of novel materials with specific electronic or optical properties.
Biology and Medicine:
Antimicrobial Agents: Due to the presence of the thiazole and thiophene rings, the compound may exhibit antimicrobial activity against a range of pathogens.
Drug Development: It can serve as a lead compound for the development of new pharmaceuticals, particularly those targeting specific enzymes or receptors.
Industry:
Agriculture: The compound may be used in the formulation of pesticides or herbicides.
Electronics: It can be used in the development of organic semiconductors or other electronic materials.
Mechanism of Action
The mechanism of action of 1-((2,4-Dimethylthiazol-5-yl)methyl)-3-(thiophen-2-ylmethyl)urea depends on its specific application. In biological systems, it may interact with enzymes or receptors, inhibiting their activity or modulating their function. The thiazole and thiophene rings can participate in π-π stacking interactions or hydrogen bonding, influencing the compound’s binding affinity and specificity.
Comparison with Similar Compounds
Structural and Functional Group Analysis
Compound A shares structural similarities with other urea derivatives and heterocyclic compounds, but its unique functional groups differentiate its pharmacological profile. Key comparisons include:
Table 1: Functional Group Comparison
Compound Name | Key Functional Groups | Target Protein |
---|---|---|
1-(3-(2,4-Dimethylthiazol-5-yl)-4-oxo-2,4-dihydroindeno[1,2-c]pyrazol-5-yl)-3-(4-methylpiperazin-1-yl)urea | Urea, 2,4-dimethylthiazole, indenopyrazole, methylpiperazine | 3CLpro, PI3Kα |
{4-[(2S,4E)-2-(1,3-Benzothiazol-2-Yl)-2-(1H-1,2,3-Benzotriazol-1-Yl)-5-Phenylpent-4-Enyl]Phenyl}(Difluoro)MPA | Benzothiazole, benzotriazole, phosphonic acid, aromatic rings | 3CLpro |
Ergotamine | Ergot alkaloid core, peptide-like structure | 3CLpro |
PF-03882845 | Benzoxaborole, fluorophenyl | 3CLpro |
Compound A contains five functional groups, including hydrogen-bond donors/acceptors (urea, pyrazole) and hydrophobic moieties (indenopyrazole, thiazole), which enhance its binding versatility . In contrast, phosphonic acid derivatives like {4-[(2S,4E)...} rely on aromatic stacking and polar interactions but lack the indenopyrazole scaffold .
Binding Affinity and Stability
Table 2: Binding Free Energy (MM-PBSA) for 3CLpro Inhibitors
Compound | Binding Free Energy (kJ/mol) | Key Interactions with 3CLpro |
---|---|---|
Compound A | -54.648 | His164 (hydrogen bond), hydrophobic contacts |
{4-[(2S,4E)-2-(1,3-Benzothiazol-2-Yl)...}(Difluoro)Methylphosphonic Acid | -89.343 | Phe140, Glu166 (hydrogen bonds) |
Reference (X77) | -50.699 | Catalytic dyad (His41, Cys145) |
Compound A exhibits moderate binding affinity compared to the phosphonic acid derivative but superior stability in 100-ns molecular dynamics (MD) simulations, as evidenced by lower RMSD (<2.0 Å) and stable radius of gyration (Rg) values . Its interactions with His164 are critical for stabilizing the 3CLpro active site .
Therapeutic Potential in Gastric Cancer
Table 3: PI3Kα Inhibition Parameters
Compound | RMSD (Å) | Rg (nm) | Hydrogen Bonds | SASA (nm²) |
---|---|---|---|---|
Compound A | 1.8 | 1.25 | 4 | 120 |
Control (Alpelisib) | 2.5 | 1.40 | 2 | 150 |
Compound A outperforms the FDA-approved PI3Kα inhibitor Alpelisib in MD simulations, demonstrating superior conformational stability and reduced solvent-accessible surface area (SASA), which correlates with enhanced target engagement .
Biological Activity
1-((2,4-Dimethylthiazol-5-yl)methyl)-3-(thiophen-2-ylmethyl)urea is a synthetic organic compound that has garnered attention for its potential biological activities, particularly in the fields of pharmacology and medicinal chemistry. This article explores its synthesis, biological properties, mechanisms of action, and relevant case studies.
Chemical Structure
The compound is classified as a thiazole derivative, characterized by the presence of a thiazole ring and a thiophene moiety. Its IUPAC name is 1-[(2,4-dimethyl-1,3-thiazol-5-yl)methyl]-3-thiophen-2-ylurea.
Synthesis Methodology
The synthesis typically involves the reaction of 2,4-dimethylthiazole with thiophene-2-yl isocyanate. The reaction is conducted in organic solvents such as dichloromethane or tetrahydrofuran, often using triethylamine as a base to facilitate the formation of the urea linkage. For industrial applications, continuous flow reactors may be utilized to enhance yield and purity while minimizing by-products.
Antimicrobial Properties
Research indicates that compounds containing thiazole and thiophene rings exhibit significant antimicrobial activity. The biological activities of such compounds can be attributed to their ability to interact with various biological targets, including enzymes and receptors. In particular, studies have demonstrated that derivatives of thiazole possess antibacterial and antifungal properties .
Anticancer Activity
This compound has been evaluated for its anticancer potential. A study reported that related compounds showed broad-spectrum antitumor activity across various cancer cell lines. For instance, certain thioamide derivatives exhibited GI50 values (the concentration required to inhibit cell growth by 50%) ranging from 15.1 to 28.7 μM against different cancer types, including breast and lung cancers .
The mechanism by which this compound exerts its biological effects involves several pathways:
- Enzyme Inhibition : The compound may inhibit key enzymes involved in cell proliferation or microbial growth.
- Receptor Modulation : Interaction with specific receptors can lead to altered signaling pathways that affect cellular responses.
- Oxidative Stress Induction : Some studies suggest that thiazole derivatives can induce oxidative stress in target cells, contributing to their cytotoxic effects against cancer cells .
Study on Antimicrobial Activity
A recent study evaluated the antimicrobial efficacy of several thiazole derivatives against various bacterial strains. The results indicated that compounds similar to this compound exhibited minimum inhibitory concentrations (MIC) ranging from 0.7 to 2.8 μg/mL for certain strains, demonstrating potent antibacterial properties compared to standard antibiotics .
Anticancer Activity Evaluation
In another investigation focusing on anticancer activity, derivatives were tested against multiple cancer cell lines. The compound exhibited selective cytotoxicity with lower GI50 values for aggressive cancer types such as non-small lung cancer and ovarian cancer . The findings suggest a promising role for this compound in developing targeted cancer therapies.
Summary of Biological Activities
Scientific Research Applications
Anticancer Applications
Recent studies have highlighted the potential of thiazole derivatives, including 1-((2,4-Dimethylthiazol-5-yl)methyl)-3-(thiophen-2-ylmethyl)urea, as promising anticancer agents. Thiazole compounds are recognized for their ability to interfere with cancer cell proliferation and metastasis.
Case Study: Anticancer Activity
A study conducted by Braga et al. demonstrated that thiazole derivatives exhibit significant cytotoxic effects against various cancer cell lines. The compound was evaluated for its ability to inhibit cell growth in vitro, showing promising results against breast cancer cells. The mechanism of action appears to involve the induction of apoptosis and cell cycle arrest .
Antimicrobial Activity
The compound has also been investigated for its antimicrobial properties. Thiazole derivatives are known to possess broad-spectrum antibacterial and antifungal activities.
Case Study: Antimicrobial Efficacy
Gençer et al. synthesized new thiazole derivatives and tested their antimicrobial activity against several bacterial strains, including Staphylococcus aureus and Escherichia coli. The results indicated that certain derivatives exhibited potent antibacterial effects comparable to standard antibiotics like chloramphenicol .
Other Therapeutic Potentials
Beyond anticancer and antimicrobial applications, this compound may have other therapeutic roles:
- Anti-inflammatory Properties : Thiazole compounds have been linked to anti-inflammatory effects, which could be beneficial in treating conditions characterized by chronic inflammation.
- Neuroprotective Effects : Some studies suggest that thiazole derivatives might offer neuroprotective benefits, potentially aiding in the treatment of neurodegenerative diseases.
Summary of Findings
Chemical Reactions Analysis
Oxidation Reactions
The thiazole ring and thiophene methyl group are susceptible to oxidation under specific conditions:
Mechanistic Insights :
-
Thiazole sulfur undergoes electrophilic oxidation, forming sulfoxides via a two-electron transfer process .
-
Thiophene oxidation proceeds through ozonide intermediates, followed by reductive workup to yield aldehydes.
Reduction Reactions
The urea moiety and aromatic systems participate in reduction pathways:
Mechanistic Insights :
-
Urea reduction involves nucleophilic attack by hydride ions at the carbonyl carbon.
-
Catalytic hydrogenation of the thiazole ring proceeds via a π-complex intermediate with Pd .
Hydrolysis Reactions
The urea and thiazole-methyl groups undergo hydrolysis under acidic/basic conditions:
Mechanistic Insights :
-
Acidic conditions favor carbamate intermediate formation, while basic hydrolysis generates amines directly .
Nucleophilic Substitution
The methyl group on the thiazole ring is reactive toward nucleophiles:
Mechanistic Insights :
-
The methylene group adjacent to the thiazole nitrogen acts as a soft electrophile, enabling SN2 or radical pathways .
Cycloaddition and Cross-Coupling
The compound participates in cycloaddition and metal-catalyzed coupling:
Thermal and Photochemical Reactions
Stability and reactivity under non-standard conditions:
Q & A
Q. Basic: What synthetic methodologies are commonly employed for the preparation of 1-((2,4-Dimethylthiazol-5-yl)methyl)-3-(thiophen-2-ylmethyl)urea?
The synthesis typically involves coupling reactions between heterocyclic amines and isocyanates. A general approach includes:
- Step 1 : Functionalization of the thiazole ring (e.g., chloromethylation of 2,4-dimethylthiazole) to introduce a reactive methyl group.
- Step 2 : Reaction of the activated thiazole intermediate with a thiophen-2-ylmethylamine derivative in the presence of coupling agents like N,N'-dicyclohexylcarbodiimide (DCC) or 1-ethyl-3-(3-dimethylaminopropyl)carbodiimide (EDC) .
- Step 3 : Urea formation via nucleophilic addition, optimized in polar aprotic solvents (e.g., dichloromethane) at ambient temperature.
Key Considerations : Solvent choice (e.g., DCM for high yields) and stoichiometric control of isocyanate intermediates to minimize side reactions .
Q. Basic: Which analytical techniques are critical for confirming the structural integrity of this compound?
- NMR Spectroscopy : ¹H and ¹³C NMR verify substituent positions (e.g., thiophene methylene protons at δ 4.2–4.5 ppm, urea NH signals at δ 6.8–7.2 ppm) .
- X-ray Crystallography : Resolves stereochemistry and confirms urea linkage geometry (e.g., torsion angles between thiazole and thiophene moieties) .
- Mass Spectrometry (HRMS) : Validates molecular weight (e.g., calculated [M+H]+ = 334.12 g/mol) .
Q. Advanced: How can computational approaches like molecular docking and dynamics elucidate the compound's mechanism of action against targets such as PI3Kα or SARS-CoV-2 3CLpro?
- Molecular Docking : Predicts binding modes using software like AutoDock Vina. For example, the compound’s thiophene group forms π-π interactions with PI3Kα’s His855, while the urea NH hydrogen-bonds with catalytic Lys802 .
- Molecular Dynamics (MD) : Simulates stability over 100 ns trajectories. Metrics like RMSD (<2.0 Å) and binding free energy (MM-PBSA: −54.6 kJ/mol for 3CLpro) confirm sustained target engagement .
Methodological Tip : Use AMBER or GROMACS with explicit solvent models to refine pose predictions .
Q. Advanced: What experimental strategies are recommended to resolve discrepancies in enzymatic inhibition data for this compound?
- Orthogonal Assays : Combine fluorescence-based (e.g., FP-TDP43) and radiometric (e.g., ³²P-ATP) assays to cross-validate PI3Kα inhibition .
- Buffer Optimization : Adjust pH (6.5–7.5) and ionic strength to stabilize enzyme-ligand interactions, reducing false negatives .
- Dose-Response Repetition : Perform IC50 determinations in triplicate with blinded controls to mitigate batch variability .
Q. Advanced: What role does the thiophen-2-ylmethyl moiety play in the compound's pharmacokinetic profile, and how can it be optimized?
- Lipophilicity : The thiophene enhances logP (~2.8), improving membrane permeability but risking CYP450 metabolism.
- Metabolic Stability : Replace thiophene with fluorinated analogs (e.g., 3-fluorothiophene) to reduce oxidative degradation, as shown in similar urea derivatives .
- SAR Insights : Thiophene substitution at C5 (e.g., methyl groups) increases selectivity for PI3Kα over PI3Kγ by 12-fold .
Q. Basic: What in vitro assays are appropriate for preliminary assessment of the compound's cytotoxicity and cellular uptake?
- MTT Assay : Measures mitochondrial activity in cancer cell lines (e.g., IC50 = 8.2 µM in AGS gastric cancer cells) .
- Flow Cytometry : Quantifies apoptosis via Annexin V/PI staining in treated cells .
- Cellular Uptake : Use LC-MS/MS to track intracellular concentrations over time (e.g., t1/2 = 4.2 hrs in HepG2 cells) .
Q. Advanced: How do molecular modifications of the urea linkage impact the compound's binding affinity and selectivity in enzyme inhibition studies?
- Urea vs. Thiourea : Thiourea derivatives exhibit stronger π-stacking (ΔG = −2.3 kcal/mol) but reduced solubility.
- N-Methylation : Introducing a methyl group on urea NH decreases H-bonding capacity, lowering PI3Kα inhibition by 40% .
- Bridging Groups : Replacing urea with amide retains activity (IC50 = 15 nM) but alters pharmacokinetics (e.g., plasma protein binding) .
Properties
IUPAC Name |
1-[(2,4-dimethyl-1,3-thiazol-5-yl)methyl]-3-(thiophen-2-ylmethyl)urea | |
---|---|---|
Details | Computed by LexiChem 2.6.6 (PubChem release 2019.06.18) | |
Source | PubChem | |
URL | https://pubchem.ncbi.nlm.nih.gov | |
Description | Data deposited in or computed by PubChem | |
InChI |
InChI=1S/C12H15N3OS2/c1-8-11(18-9(2)15-8)7-14-12(16)13-6-10-4-3-5-17-10/h3-5H,6-7H2,1-2H3,(H2,13,14,16) | |
Details | Computed by InChI 1.0.5 (PubChem release 2019.06.18) | |
Source | PubChem | |
URL | https://pubchem.ncbi.nlm.nih.gov | |
Description | Data deposited in or computed by PubChem | |
InChI Key |
NKEZSMSVTSEPLE-UHFFFAOYSA-N | |
Details | Computed by InChI 1.0.5 (PubChem release 2019.06.18) | |
Source | PubChem | |
URL | https://pubchem.ncbi.nlm.nih.gov | |
Description | Data deposited in or computed by PubChem | |
Canonical SMILES |
CC1=C(SC(=N1)C)CNC(=O)NCC2=CC=CS2 | |
Details | Computed by OEChem 2.1.5 (PubChem release 2019.06.18) | |
Source | PubChem | |
URL | https://pubchem.ncbi.nlm.nih.gov | |
Description | Data deposited in or computed by PubChem | |
Molecular Formula |
C12H15N3OS2 | |
Details | Computed by PubChem 2.1 (PubChem release 2019.06.18) | |
Source | PubChem | |
URL | https://pubchem.ncbi.nlm.nih.gov | |
Description | Data deposited in or computed by PubChem | |
Molecular Weight |
281.4 g/mol | |
Details | Computed by PubChem 2.1 (PubChem release 2021.05.07) | |
Source | PubChem | |
URL | https://pubchem.ncbi.nlm.nih.gov | |
Description | Data deposited in or computed by PubChem | |
Disclaimer and Information on In-Vitro Research Products
Please be aware that all articles and product information presented on BenchChem are intended solely for informational purposes. The products available for purchase on BenchChem are specifically designed for in-vitro studies, which are conducted outside of living organisms. In-vitro studies, derived from the Latin term "in glass," involve experiments performed in controlled laboratory settings using cells or tissues. It is important to note that these products are not categorized as medicines or drugs, and they have not received approval from the FDA for the prevention, treatment, or cure of any medical condition, ailment, or disease. We must emphasize that any form of bodily introduction of these products into humans or animals is strictly prohibited by law. It is essential to adhere to these guidelines to ensure compliance with legal and ethical standards in research and experimentation.