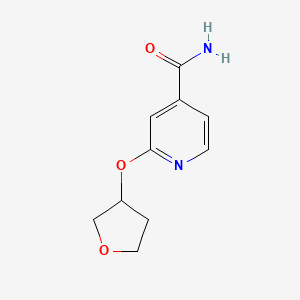
2-(oxolan-3-yloxy)pyridine-4-carboxamide
- Click on QUICK INQUIRY to receive a quote from our team of experts.
- With the quality product at a COMPETITIVE price, you can focus more on your research.
Overview
Description
2-(oxolan-3-yloxy)pyridine-4-carboxamide is a chemical compound that has garnered interest in various fields of scientific research due to its unique structural properties and potential applications. This compound features a tetrahydrofuran ring attached to an isonicotinamide moiety, making it a versatile molecule for various chemical reactions and applications.
Preparation Methods
Synthetic Routes and Reaction Conditions
The synthesis of 2-(oxolan-3-yloxy)pyridine-4-carboxamide typically involves the reaction of tetrahydrofuran derivatives with isonicotinamide under specific conditions. One common method involves the use of tetrahydrofuran-3-ol and isonicotinoyl chloride in the presence of a base such as triethylamine. The reaction is carried out in an organic solvent like dichloromethane at room temperature, leading to the formation of the desired product .
Industrial Production Methods
Industrial production of this compound may involve similar synthetic routes but on a larger scale. The use of continuous flow reactors and optimized reaction conditions can enhance the yield and purity of the compound. Catalysts and automated systems are often employed to ensure consistent production quality .
Chemical Reactions Analysis
Types of Reactions
2-(oxolan-3-yloxy)pyridine-4-carboxamide undergoes various types of chemical reactions, including:
Oxidation: The compound can be oxidized using oxidizing agents like potassium permanganate or hydrogen peroxide.
Reduction: Reduction reactions can be carried out using reducing agents such as lithium aluminum hydride.
Substitution: Nucleophilic substitution reactions are common, where the tetrahydrofuran ring can be substituted with other functional groups.
Common Reagents and Conditions
Oxidation: Potassium permanganate in an acidic medium.
Reduction: Lithium aluminum hydride in anhydrous ether.
Substitution: Nucleophiles like sodium azide or sodium methoxide in polar solvents.
Major Products Formed
The major products formed from these reactions depend on the specific reagents and conditions used. For example, oxidation may yield carboxylic acids, while reduction can produce alcohols. Substitution reactions can introduce various functional groups into the tetrahydrofuran ring .
Scientific Research Applications
2-(oxolan-3-yloxy)pyridine-4-carboxamide has a wide range of applications in scientific research:
Chemistry: Used as a building block for the synthesis of more complex molecules.
Biology: Investigated for its potential as a bioactive compound with antimicrobial properties.
Medicine: Explored for its potential use in drug development, particularly in targeting specific enzymes or receptors.
Industry: Utilized in the production of specialty chemicals and materials.
Mechanism of Action
The mechanism of action of 2-(oxolan-3-yloxy)pyridine-4-carboxamide involves its interaction with specific molecular targets. The compound can bind to enzymes or receptors, modulating their activity. The tetrahydrofuran ring and isonicotinamide moiety play crucial roles in its binding affinity and specificity. Pathways involved may include inhibition of enzyme activity or receptor signaling .
Comparison with Similar Compounds
Similar Compounds
- 2-((Tetrahydrofuran-3-yl)oxy)nicotinic acid
- N-Methoxy-2-((tetrahydrofuran-3-yl)oxy)isonicotinamide
- N,N-Dimethyl-2-((tetrahydrofuran-3-yl)oxy)isonicotinamide
Uniqueness
2-(oxolan-3-yloxy)pyridine-4-carboxamide stands out due to its unique combination of the tetrahydrofuran ring and isonicotinamide moiety. This structural feature imparts distinct chemical reactivity and biological activity, making it a valuable compound for various applications .
Biological Activity
2-(Oxolan-3-yloxy)pyridine-4-carboxamide is a complex organic compound that features a pyridine ring and an oxolan moiety. This unique structure contributes to its potential biological activities, including antimicrobial and antitumor properties. The compound's molecular formula is C11H12N2O3, with an approximate molecular weight of 220.22 g/mol.
Structural Characteristics
The structure of this compound includes:
- A pyridine-4-carboxamide core.
- An oxolan-3-yloxy group that enhances solubility and reactivity.
The biological activity of this compound is primarily attributed to its interaction with specific molecular targets, such as enzymes and receptors. The compound may modulate these targets through binding interactions, leading to alterations in cellular processes. The mechanism can involve:
- Hydrogen bonding and hydrophobic interactions , crucial for its biological efficacy.
Antimicrobial Properties
Research indicates that derivatives of this compound exhibit effectiveness against various bacterial strains, suggesting potential applications in antibiotic development. For instance, certain derivatives have shown significant activity against resistant strains, which is critical in the context of rising antibiotic resistance.
Antitumor Activity
Preliminary studies have indicated that this compound may possess antitumor properties. In vitro assays demonstrated cytotoxic effects on cancer cell lines, although further studies are needed to elucidate the exact mechanisms and efficacy in vivo.
Structure-Activity Relationship (SAR)
A comprehensive SAR analysis has been conducted to understand how structural modifications affect biological activity. Key findings include:
- Compounds with increased lipophilicity tend to exhibit enhanced potency against specific targets.
- Substituents on the pyridine ring significantly influence the binding affinity and selectivity for biological targets.
Compound | Structural Features | Biological Activity | EC50 (nM) |
---|---|---|---|
Compound A | Pyridine core with oxolane | Antimicrobial | 150 |
Compound B | Pyridine core with additional methyl group | Antitumor | 75 |
Compound C | Pyridine core without oxolane | Low activity | >1000 |
Case Studies
-
Antimicrobial Efficacy Study
- A study evaluated the antimicrobial activity of various derivatives against E. coli and Staphylococcus aureus.
- Results indicated that certain derivatives exhibited an EC50 of approximately 150 nM against E. coli, showcasing their potential as new antimicrobial agents.
-
Antitumor Activity Assessment
- In vitro tests on breast cancer cell lines revealed that this compound induced apoptosis at concentrations as low as 75 nM.
- Further investigation into the signaling pathways involved showed activation of caspase cascades, confirming its role in promoting cell death in tumor cells.
Properties
IUPAC Name |
2-(oxolan-3-yloxy)pyridine-4-carboxamide |
Source
|
---|---|---|
Details | Computed by Lexichem TK 2.7.0 (PubChem release 2021.05.07) | |
Source | PubChem | |
URL | https://pubchem.ncbi.nlm.nih.gov | |
Description | Data deposited in or computed by PubChem | |
InChI |
InChI=1S/C10H12N2O3/c11-10(13)7-1-3-12-9(5-7)15-8-2-4-14-6-8/h1,3,5,8H,2,4,6H2,(H2,11,13) |
Source
|
Details | Computed by InChI 1.0.6 (PubChem release 2021.05.07) | |
Source | PubChem | |
URL | https://pubchem.ncbi.nlm.nih.gov | |
Description | Data deposited in or computed by PubChem | |
InChI Key |
CKLZYHRCGAABDQ-UHFFFAOYSA-N |
Source
|
Details | Computed by InChI 1.0.6 (PubChem release 2021.05.07) | |
Source | PubChem | |
URL | https://pubchem.ncbi.nlm.nih.gov | |
Description | Data deposited in or computed by PubChem | |
Canonical SMILES |
C1COCC1OC2=NC=CC(=C2)C(=O)N |
Source
|
Details | Computed by OEChem 2.3.0 (PubChem release 2021.05.07) | |
Source | PubChem | |
URL | https://pubchem.ncbi.nlm.nih.gov | |
Description | Data deposited in or computed by PubChem | |
Molecular Formula |
C10H12N2O3 |
Source
|
Details | Computed by PubChem 2.1 (PubChem release 2021.05.07) | |
Source | PubChem | |
URL | https://pubchem.ncbi.nlm.nih.gov | |
Description | Data deposited in or computed by PubChem | |
Molecular Weight |
208.21 g/mol |
Source
|
Details | Computed by PubChem 2.1 (PubChem release 2021.05.07) | |
Source | PubChem | |
URL | https://pubchem.ncbi.nlm.nih.gov | |
Description | Data deposited in or computed by PubChem | |
Disclaimer and Information on In-Vitro Research Products
Please be aware that all articles and product information presented on BenchChem are intended solely for informational purposes. The products available for purchase on BenchChem are specifically designed for in-vitro studies, which are conducted outside of living organisms. In-vitro studies, derived from the Latin term "in glass," involve experiments performed in controlled laboratory settings using cells or tissues. It is important to note that these products are not categorized as medicines or drugs, and they have not received approval from the FDA for the prevention, treatment, or cure of any medical condition, ailment, or disease. We must emphasize that any form of bodily introduction of these products into humans or animals is strictly prohibited by law. It is essential to adhere to these guidelines to ensure compliance with legal and ethical standards in research and experimentation.