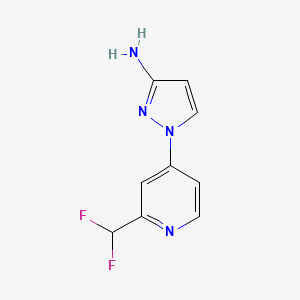
Nc1ccn(n1)-c1ccnc(c1)C(F)F
- Click on QUICK INQUIRY to receive a quote from our team of experts.
- With the quality product at a COMPETITIVE price, you can focus more on your research.
Overview
Description
The compound with the chemical structure “Nc1ccn(n1)-c1ccnc(c1)C(F)F” is a heterocyclic aromatic compound. It contains a pyridine ring and a difluoromethyl group, which are known for their significant roles in medicinal chemistry and material science. The presence of nitrogen atoms in the ring structure often imparts unique electronic properties, making such compounds valuable in various applications.
Preparation Methods
Synthetic Routes and Reaction Conditions
The synthesis of “Nc1ccn(n1)-c1ccnc(c1)C(F)F” typically involves the formation of the pyridine ring followed by the introduction of the difluoromethyl group. One common method is the cyclization of appropriate precursors under acidic or basic conditions. The difluoromethyl group can be introduced using reagents like difluoromethyl iodide or difluoromethyl sulfone under specific conditions such as the presence of a base or a catalyst.
Industrial Production Methods
Industrial production of this compound may involve large-scale cyclization reactions using automated reactors to ensure consistency and yield
Chemical Reactions Analysis
Types of Reactions
The compound “Nc1ccn(n1)-c1ccnc(c1)C(F)F” can undergo various chemical reactions, including:
Oxidation: The nitrogen atoms in the pyridine ring can be oxidized to form N-oxides.
Reduction: The compound can be reduced to form different hydrogenated derivatives.
Common Reagents and Conditions
Oxidation: Reagents like hydrogen peroxide or m-chloroperbenzoic acid (mCPBA) under mild conditions.
Reduction: Catalytic hydrogenation using palladium on carbon (Pd/C) or lithium aluminum hydride (LiAlH4).
Substitution: Halogenating agents like N-bromosuccinimide (NBS) or nucleophiles like amines and thiols.
Major Products
The major products formed from these reactions include N-oxides, hydrogenated derivatives, and various substituted pyridine derivatives, depending on the specific reagents and conditions used.
Scientific Research Applications
The compound “Nc1ccn(n1)-c1ccnc(c1)C(F)F” has diverse applications in scientific research:
Chemistry: Used as a building block for the synthesis of more complex molecules.
Biology: Investigated for its potential as a ligand in biochemical assays.
Medicine: Explored for its pharmacological properties, including potential use as an antimicrobial or anticancer agent.
Industry: Utilized in the development of advanced materials with specific electronic properties.
Mechanism of Action
The mechanism by which “Nc1ccn(n1)-c1ccnc(c1)C(F)F” exerts its effects often involves interactions with specific molecular targets. The nitrogen atoms in the pyridine ring can form hydrogen bonds or coordinate with metal ions, influencing various biochemical pathways. The difluoromethyl group can enhance the compound’s lipophilicity, improving its ability to cross cell membranes and interact with intracellular targets.
Comparison with Similar Compounds
Similar Compounds
Pyridine: A simpler analog without the difluoromethyl group.
2,6-Difluoropyridine: Contains fluorine atoms but lacks the additional nitrogen atoms.
Nicotinamide: Contains a similar pyridine ring but with an amide group.
Uniqueness
The unique combination of the pyridine ring and the difluoromethyl group in “Nc1ccn(n1)-c1ccnc(c1)C(F)F” imparts distinct electronic properties and reactivity, making it valuable for specific applications where other similar compounds may not be as effective.
This detailed article provides a comprehensive overview of the compound “this compound,” covering its synthesis, reactions, applications, mechanism of action, and comparison with similar compounds
Properties
IUPAC Name |
1-[2-(difluoromethyl)pyridin-4-yl]pyrazol-3-amine |
Source
|
---|---|---|
Details | Computed by Lexichem TK 2.7.0 (PubChem release 2021.05.07) | |
Source | PubChem | |
URL | https://pubchem.ncbi.nlm.nih.gov | |
Description | Data deposited in or computed by PubChem | |
InChI |
InChI=1S/C9H8F2N4/c10-9(11)7-5-6(1-3-13-7)15-4-2-8(12)14-15/h1-5,9H,(H2,12,14) |
Source
|
Details | Computed by InChI 1.0.6 (PubChem release 2021.05.07) | |
Source | PubChem | |
URL | https://pubchem.ncbi.nlm.nih.gov | |
Description | Data deposited in or computed by PubChem | |
InChI Key |
GNJYUJWFBIUJMB-UHFFFAOYSA-N |
Source
|
Details | Computed by InChI 1.0.6 (PubChem release 2021.05.07) | |
Source | PubChem | |
URL | https://pubchem.ncbi.nlm.nih.gov | |
Description | Data deposited in or computed by PubChem | |
Canonical SMILES |
C1=CN=C(C=C1N2C=CC(=N2)N)C(F)F |
Source
|
Details | Computed by OEChem 2.3.0 (PubChem release 2021.05.07) | |
Source | PubChem | |
URL | https://pubchem.ncbi.nlm.nih.gov | |
Description | Data deposited in or computed by PubChem | |
Molecular Formula |
C9H8F2N4 |
Source
|
Details | Computed by PubChem 2.1 (PubChem release 2021.05.07) | |
Source | PubChem | |
URL | https://pubchem.ncbi.nlm.nih.gov | |
Description | Data deposited in or computed by PubChem | |
Molecular Weight |
210.18 g/mol |
Source
|
Details | Computed by PubChem 2.1 (PubChem release 2021.05.07) | |
Source | PubChem | |
URL | https://pubchem.ncbi.nlm.nih.gov | |
Description | Data deposited in or computed by PubChem | |
Disclaimer and Information on In-Vitro Research Products
Please be aware that all articles and product information presented on BenchChem are intended solely for informational purposes. The products available for purchase on BenchChem are specifically designed for in-vitro studies, which are conducted outside of living organisms. In-vitro studies, derived from the Latin term "in glass," involve experiments performed in controlled laboratory settings using cells or tissues. It is important to note that these products are not categorized as medicines or drugs, and they have not received approval from the FDA for the prevention, treatment, or cure of any medical condition, ailment, or disease. We must emphasize that any form of bodily introduction of these products into humans or animals is strictly prohibited by law. It is essential to adhere to these guidelines to ensure compliance with legal and ethical standards in research and experimentation.