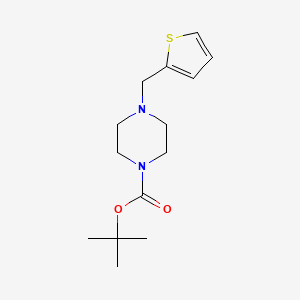
Tert-butyl 4-(thiophen-2-ylmethyl)piperazine-1-carboxylate
- Click on QUICK INQUIRY to receive a quote from our team of experts.
- With the quality product at a COMPETITIVE price, you can focus more on your research.
Overview
Description
Tert-butyl 4-(thiophen-2-ylmethyl)piperazine-1-carboxylate: is a chemical compound with the molecular formula C14H22N2O2S and a molecular weight of 282.41 g/mol . It is a versatile small molecule scaffold used in various research and industrial applications .
Mechanism of Action
Target of Action
The primary targets of “Tert-butyl 4-(thiophen-2-ylmethyl)piperazine-1-carboxylate” are currently unknown. This compound is a derivative of piperazine, which is known to interact with a variety of targets, including neurotransmitter receptors
Mode of Action
Piperazine derivatives are known to interact with their targets in a variety of ways, often acting as agonists or antagonists . The thiophen-2-ylmethyl group may influence the compound’s interaction with its targets, potentially altering receptor binding or signal transduction .
Biochemical Pathways
Given the compound’s structural similarity to piperazine, it may influence pathways involving neurotransmission
Pharmacokinetics
Piperazine derivatives are generally well absorbed and widely distributed in the body . The tert-butyl group may influence the compound’s metabolic stability, while the thiophen-2-ylmethyl group may affect its distribution
Result of Action
Based on its structural similarity to piperazine, it may influence neurotransmission, potentially leading to changes in neuronal activity
Action Environment
Environmental factors can significantly influence the action, efficacy, and stability of “this compound”. Factors such as temperature, pH, and the presence of other molecules can affect the compound’s stability and interaction with its targets
Preparation Methods
Synthetic Routes and Reaction Conditions: The synthesis of tert-butyl 4-(thiophen-2-ylmethyl)piperazine-1-carboxylate typically involves the reaction of thiophen-2-ylmethylamine with tert-butyl 4-piperazinecarboxylate under controlled conditions . The reaction is usually carried out in the presence of a base such as triethylamine and a solvent like dichloromethane at room temperature .
Industrial Production Methods: Industrial production methods for this compound are similar to laboratory synthesis but are scaled up to accommodate larger quantities. The reaction conditions are optimized for efficiency and yield, often involving continuous flow reactors and automated systems to ensure consistency and quality .
Chemical Reactions Analysis
Types of Reactions: Tert-butyl 4-(thiophen-2-ylmethyl)piperazine-1-carboxylate undergoes various chemical reactions, including:
Oxidation: The thiophene ring can be oxidized to form sulfoxides or sulfones under appropriate conditions.
Reduction: The compound can be reduced to form different derivatives, depending on the reducing agents used.
Substitution: The piperazine ring can undergo nucleophilic substitution reactions, leading to the formation of various substituted derivatives.
Common Reagents and Conditions:
Oxidation: Common oxidizing agents include hydrogen peroxide and m-chloroperbenzoic acid.
Reduction: Reducing agents such as lithium aluminum hydride or sodium borohydride are commonly used.
Substitution: Nucleophiles like alkyl halides or acyl chlorides are used in substitution reactions.
Major Products Formed: The major products formed from these reactions depend on the specific reagents and conditions used. For example, oxidation of the thiophene ring can yield sulfoxides or sulfones, while substitution reactions can produce a variety of substituted piperazine derivatives .
Scientific Research Applications
Tert-butyl 4-(thiophen-2-ylmethyl)piperazine-1-carboxylate has several scientific research applications, including:
Comparison with Similar Compounds
Tert-butyl 4-(piperidin-4-ylmethyl)piperazine-1-carboxylate: This compound has a similar structure but with a piperidine ring instead of a thiophene ring.
Tert-butyl 4-(benzo[b]thiophen-4-yl)piperazine-1-carboxylate: This compound features a benzo[b]thiophene ring, providing different chemical properties and reactivity.
Uniqueness: Tert-butyl 4-(thiophen-2-ylmethyl)piperazine-1-carboxylate is unique due to the presence of the thiophene ring, which imparts specific electronic and steric properties. This uniqueness makes it valuable in the synthesis of novel compounds and in various research applications .
Properties
IUPAC Name |
tert-butyl 4-(thiophen-2-ylmethyl)piperazine-1-carboxylate |
Source
|
---|---|---|
Details | Computed by Lexichem TK 2.7.0 (PubChem release 2021.05.07) | |
Source | PubChem | |
URL | https://pubchem.ncbi.nlm.nih.gov | |
Description | Data deposited in or computed by PubChem | |
InChI |
InChI=1S/C14H22N2O2S/c1-14(2,3)18-13(17)16-8-6-15(7-9-16)11-12-5-4-10-19-12/h4-5,10H,6-9,11H2,1-3H3 |
Source
|
Details | Computed by InChI 1.0.6 (PubChem release 2021.05.07) | |
Source | PubChem | |
URL | https://pubchem.ncbi.nlm.nih.gov | |
Description | Data deposited in or computed by PubChem | |
InChI Key |
XCORMTCZQFGXFU-UHFFFAOYSA-N |
Source
|
Details | Computed by InChI 1.0.6 (PubChem release 2021.05.07) | |
Source | PubChem | |
URL | https://pubchem.ncbi.nlm.nih.gov | |
Description | Data deposited in or computed by PubChem | |
Canonical SMILES |
CC(C)(C)OC(=O)N1CCN(CC1)CC2=CC=CS2 |
Source
|
Details | Computed by OEChem 2.3.0 (PubChem release 2021.05.07) | |
Source | PubChem | |
URL | https://pubchem.ncbi.nlm.nih.gov | |
Description | Data deposited in or computed by PubChem | |
Molecular Formula |
C14H22N2O2S |
Source
|
Details | Computed by PubChem 2.1 (PubChem release 2021.05.07) | |
Source | PubChem | |
URL | https://pubchem.ncbi.nlm.nih.gov | |
Description | Data deposited in or computed by PubChem | |
Molecular Weight |
282.40 g/mol |
Source
|
Details | Computed by PubChem 2.1 (PubChem release 2021.05.07) | |
Source | PubChem | |
URL | https://pubchem.ncbi.nlm.nih.gov | |
Description | Data deposited in or computed by PubChem | |
Disclaimer and Information on In-Vitro Research Products
Please be aware that all articles and product information presented on BenchChem are intended solely for informational purposes. The products available for purchase on BenchChem are specifically designed for in-vitro studies, which are conducted outside of living organisms. In-vitro studies, derived from the Latin term "in glass," involve experiments performed in controlled laboratory settings using cells or tissues. It is important to note that these products are not categorized as medicines or drugs, and they have not received approval from the FDA for the prevention, treatment, or cure of any medical condition, ailment, or disease. We must emphasize that any form of bodily introduction of these products into humans or animals is strictly prohibited by law. It is essential to adhere to these guidelines to ensure compliance with legal and ethical standards in research and experimentation.