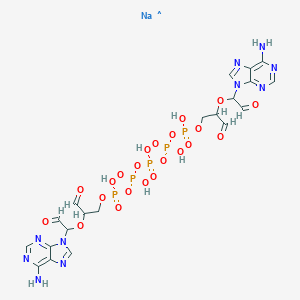
P1,P5-DI(Adenosine-5/') pentaphosphate*PE riodate oxi
Overview
Description
P1,P5-Di(Adenosine-5') pentaphosphate*periodate oxi (CAS: 107148-01-6) is a chemically modified bisubstrate analog derived from P1,P5-di(adenosine-5') pentaphosphate (Ap5A). The compound undergoes periodate oxidation, which oxidizes the 2' and 3' hydroxyl groups of adenosine's ribose moieties to form dialdehyde derivatives . Ap5A itself is a potent inhibitor of adenylate kinases (AKs), mimicking the transition state of ATP and AMP binding . The oxidized form is utilized in biochemical assays requiring stable enzyme inhibition or structural studies .
Preparation Methods
Synthetic Routes and Reaction Conditions: The synthesis of P1,P5-DI(Adenosine-5’) pentaphosphate involves a two-step process:
Formation of Adenosine 5’-tetraphosphate (P4A): This step involves the reaction of ATP with trimetaphosphate (P3) under optimal pH conditions ranging from 7.5 to 8.5.
Conversion to P1,P5-DI(Adenosine-5’) pentaphosphate: The P4A formed in the first step is then converted to P1,P5-DI(Adenosine-5’) pentaphosphate. The synthesis is modulated by metal ions to achieve the desired product.
Industrial Production Methods: While specific industrial production methods are not detailed, the synthesis typically involves controlled reaction conditions to ensure high purity and yield. The compound is available in various forms, including pentasodium and trilithium salts .
Chemical Reactions Analysis
Types of Reactions: P1,P5-DI(Adenosine-5’) pentaphosphate undergoes several types of chemical reactions, including:
Oxidation: The compound can be oxidized under specific conditions, leading to the formation of various oxidized products.
Reduction: Reduction reactions can also occur, although specific conditions and reagents are required.
Substitution: The compound can undergo substitution reactions, particularly involving its phosphate groups.
Common Reagents and Conditions:
Oxidizing Agents: Common oxidizing agents include periodate and other strong oxidizers.
Reducing Agents: Reducing agents such as sodium borohydride can be used under controlled conditions.
Substitution Reagents: Various nucleophiles can be used for substitution reactions, depending on the desired product.
Major Products: The major products formed from these reactions include various phosphorylated derivatives and oxidized forms of the compound .
Scientific Research Applications
Chemical Properties and Reactions
Ap5A undergoes various chemical reactions, including oxidation, reduction, and substitution. These reactions can yield phosphorylated derivatives and oxidized forms that are crucial for its biological activity. Common reagents used in these reactions include periodate as an oxidizing agent and sodium borohydride as a reducing agent .
Chemistry
Ap5A is utilized as a reagent in phosphorylation studies and energy transfer investigations. Its ability to participate in various chemical reactions makes it valuable for synthesizing other nucleotide analogs.
Biology
In biological research, Ap5A is primarily studied for its role as an inhibitor of adenylate kinase (ADK). This enzyme is responsible for the rephosphorylation of AMP to ADP, consuming ATP in the process. By inhibiting ADK, Ap5A allows researchers to investigate energy metabolism without interference from ADK activity .
Case Study: Fragmented Sarcoplasmic Reticulum
Research involving fragmented sarcoplasmic reticulum from bullfrog skeletal muscle demonstrated that Ap5A effectively inhibits adenylate kinase activity without affecting calcium uptake or ATPase activity. This property makes it an essential tool for studying calcium movement and ATP-ADP exchange reactions .
Medicine
Ap5A has potential therapeutic applications due to its modulatory effects on various biological activities. For instance, it has been shown to increase the open probability of ryanodine receptor gates in cardiac muscle at picomolar to nanomolar concentrations, suggesting its role in cardiac signaling pathways .
Therapeutic Potential:
- Cardiac Function: Enhances calcium release from the sarcoplasmic reticulum.
- Neurotransmission: Modulates purinergic receptors, influencing neurotransmitter release.
Mechanism of Action
The mechanism of action of P1,P5-DI(Adenosine-5’) pentaphosphate involves its interaction with purinergic receptors in the nervous system . It activates 5’-nucleotidase and inhibits adenosine kinase activity in vitro . The compound is metabolized by soluble enzymes in the blood plasma and by membrane-bound ectoenzymes of various cell types, including endothelial and smooth muscle cells .
Comparison with Similar Compounds
Structural and Functional Differences
Diadenosine Polyphosphates (ApₙA): Ap5A belongs to the ApₙA family, where "n" denotes the number of phosphate groups. Key analogs include:
- Ap5A vs. Ap4A/Ap3A : Ap5A’s longer phosphate chain confers higher specificity for AKs, particularly mitochondrial isoforms, while shorter analogs like Ap4A exhibit broader activity across pyrophosphatases .
- Ap5A*Periodate oxi vs.
Non-Hydrolysable ATP Analogs (e.g., AMP-PNP):
- AMP-PNP mimics ATP but lacks hydrolyzable γ-phosphate.
Enzymatic Inhibition Profiles
- Adenylate Kinases (AKs) :
- Nucleotide Pyrophosphatases :
Key Research Findings
Ap5A-Induced Conformational Changes :
- Ap5A binds AK with 10-fold higher affinity than ATP, shifting domain closure equilibria at lower concentrations .
- Mutations in AK’s LID domain reduce Ap5A binding entropy, highlighting its role in stabilizing catalytic states .
Species-Specific Inhibition: Trichomonas vaginalis hydrogenosomal AK is inhibited by Ap5A (Ki ~1 µM), resembling mitochondrial isoforms .
Periodate Oxidation Impact :
- Aldehyde formation may enable Schiff base formation with lysine residues, though this remains untested in kinetic assays .
Biological Activity
P1,P5-Di(adenosine-5') pentaphosphate (Ap5A) is a diadenosine polyphosphate with significant biological activity, particularly as an inhibitor of adenylate kinase. This article explores its synthesis, mechanisms of action, physiological effects, and potential applications in various biochemical contexts.
Chemical Structure and Synthesis
Ap5A has a unique tail-to-tail dimer structure, synthesized through a two-step process starting from ATP and trimeta-phosphate. The optimal pH for its synthesis ranges from 7.5 to 8.5 and is influenced by the presence of metal ions . The molecular formula for Ap5A is , and it can exist in various salt forms, including pentasodium and trilithium salts.
Ap5A primarily functions as an adenylate kinase inhibitor . It exhibits competitive inhibition against adenylate kinase, which is crucial in cellular energy metabolism by catalyzing the conversion between ATP and ADP . The inhibitory effects are observed at concentrations as low as 2 µM, making it a potent tool for studying metabolic pathways without interference from adenylate kinase activity .
Biochemical Actions
- Inhibition of Adenylate Kinase : Ap5A inhibits adenylate kinase activity without affecting other enzymes such as hexokinase or phosphofructokinase at similar concentrations .
- Calcium Uptake : In studies involving fragmented sarcoplasmic reticulum from bullfrog skeletal muscle, Ap5A did not promote calcium uptake but inhibited adenylate kinase activity, suggesting its role in modulating calcium homeostasis indirectly .
- Ryanodine Receptor Modulation : In cardiac muscle tissues, Ap5A increases the open probability of ryanodine receptor gates at pico- to nanomolar concentrations, indicating its potential role in cardiac excitation-contraction coupling .
Physiological Effects
Ap5A is metabolized by various ectoenzymes present in endothelial and smooth muscle cells. Its biological effects extend across multiple tissues:
- Nervous System : It acts through purinergic receptors and influences neurotransmitter release and neuronal excitability .
- Cardiac Function : As mentioned, it enhances ryanodine receptor activity, which is critical for calcium-induced calcium release during cardiac muscle contraction .
- Skeletal Muscle : By inhibiting adenylate kinase, it can be used to study energy metabolism in muscle tissues under various physiological conditions .
Case Studies and Research Findings
Several studies have highlighted the diverse applications and effects of Ap5A:
Applications in Research
Ap5A's unique properties make it valuable in various experimental setups:
- Metabolic Studies : Used to dissect metabolic pathways by selectively inhibiting adenylate kinase.
- Cardiac Physiology : Investigating calcium signaling mechanisms in cardiac tissues.
- Neuroscience : Exploring neurotransmitter dynamics through purinergic signaling pathways.
Q & A
Basic Research Questions
Q. What are the critical considerations for synthesizing and purifying P1,P5-Di(adenosine-5') pentaphosphate periodate-oxidized derivatives?
- Methodological Answer: Synthesis typically involves periodate oxidation of adenosine residues to generate reactive aldehyde groups, followed by crosslinking via phosphate bridges. Purification requires ion-exchange chromatography (e.g., DEAE-Sephadex) to separate unreacted nucleotides, with confirmation via reversed-phase HPLC and mass spectrometry. Stability during synthesis is pH-sensitive; reactions should be conducted at neutral pH to prevent hydrolysis of the phosphate backbone .
Q. How should P1,P5-Di(adenosine-5') pentaphosphate periodate-oxidized sodium salt be stored to maintain stability?
- Methodological Answer: Store at –20°C in anhydrous, lyophilized form to prevent hydrolysis. Reconstitute in alkaline buffers (pH 7.5–8.5) for short-term use, as acidic conditions accelerate degradation. Avoid repeated freeze-thaw cycles, which disrupt the periodate-oxidized ribose structure .
Q. What analytical techniques are most reliable for characterizing the periodate-oxidized adenosine moieties in this compound?
- Methodological Answer: Use P-NMR to confirm phosphate linkage integrity and H-NMR to identify aldehyde peaks (δ 9–10 ppm) from oxidized ribose. UV spectroscopy at 260 nm quantifies adenosine content, while MALDI-TOF MS validates molecular weight (expected ~1,200–1,300 Da) .
Advanced Research Questions
Q. How does P1,P5-Di(adenosine-5') pentaphosphate periodate-oxidized sodium salt act as a competitive inhibitor in ATP-dependent enzymatic systems?
- Methodological Answer: The compound mimics ATP’s phosphate backbone, binding to ATPase/kinase active sites. Kinetic assays (e.g., Michaelis-Menten with varying ATP concentrations) reveal non-competitive inhibition patterns due to irreversible aldehyde-mediated crosslinking with lysine residues in enzymes. Use stopped-flow fluorimetry to monitor real-time inhibition .
Q. What experimental designs address contradictions in reported binding affinities of this compound for AMP-activated protein kinase (AMPK)?
- Methodological Answer: Discrepancies arise from variations in oxidation stoichiometry. Standardize synthesis protocols (e.g., molar ratio of periodate:adenosine) and validate purity via HPLC. Use surface plasmon resonance (SPR) with immobilized AMPK to measure binding kinetics under controlled redox conditions .
Q. Can this compound serve as a crosslinker in studying protein-nucleotide interactions? What are the optimization parameters?
- Methodological Answer: The aldehyde groups enable Schiff base formation with primary amines (e.g., lysine). Optimize crosslinking by adjusting pH (8.0–9.0) and incubation time (30–60 min). Quench unreacted aldehydes with Tris buffer. Validate via SDS-PAGE and Western blotting using anti-adenosine antibodies .
Q. How does periodate oxidation impact the compound’s role in nucleotide recycling pathways?
- Methodological Answer: Oxidation disrupts ribose ring structure, rendering it resistant to phosphorylases (e.g., purine nucleoside phosphorylase). Use C-labeled derivatives in cell lysate assays to track metabolic fate. Compare degradation rates with non-oxidized controls via TLC or LC-MS .
Q. Technical Challenges & Data Interpretation
Q. How to resolve interference from residual periodate in enzymatic assays?
- Methodological Answer: Residual periodate oxidizes thiol groups, confounding results. Add sodium bisulfite (1 mM) to quench excess periodate pre-assay. Validate by measuring free thiols (Ellman’s reagent) in control reactions without the compound .
Q. Why do discrepancies arise in reported IC values for this compound in kinase inhibition studies?
- Methodological Answer: Variations stem from differences in enzyme isoforms (e.g., AMPK α1 vs. α2) and assay buffers (divalent cation concentrations affect binding). Standardize assays using recombinant enzymes and include EDTA-free controls. Perform dose-response curves with at least 10 concentration points .
Q. Safety & Compliance
Q. What safety protocols are critical when handling periodate-oxidized adenosine derivatives?
Properties
InChI |
InChI=1S/C20H25N10O22P5.Na/c21-17-15-19(25-7-23-17)29(9-27-15)13(3-33)47-11(1-31)5-45-53(35,36)49-55(39,40)51-57(43,44)52-56(41,42)50-54(37,38)46-6-12(2-32)48-14(4-34)30-10-28-16-18(22)24-8-26-20(16)30;/h1-4,7-14H,5-6H2,(H,35,36)(H,37,38)(H,39,40)(H,41,42)(H,43,44)(H2,21,23,25)(H2,22,24,26); | |
---|---|---|
Source | PubChem | |
URL | https://pubchem.ncbi.nlm.nih.gov | |
Description | Data deposited in or computed by PubChem | |
InChI Key |
GLJPHNVVXPHWKJ-UHFFFAOYSA-N | |
Source | PubChem | |
URL | https://pubchem.ncbi.nlm.nih.gov | |
Description | Data deposited in or computed by PubChem | |
Canonical SMILES |
C1=NC(=C2C(=N1)N(C=N2)C(C=O)OC(COP(=O)(O)OP(=O)(O)OP(=O)(O)OP(=O)(O)OP(=O)(O)OCC(C=O)OC(C=O)N3C=NC4=C(N=CN=C43)N)C=O)N.[Na] | |
Source | PubChem | |
URL | https://pubchem.ncbi.nlm.nih.gov | |
Description | Data deposited in or computed by PubChem | |
Molecular Formula |
C20H25N10NaO22P5 | |
Source | PubChem | |
URL | https://pubchem.ncbi.nlm.nih.gov | |
Description | Data deposited in or computed by PubChem | |
DSSTOX Substance ID |
DTXSID60585141 | |
Record name | PUBCHEM_16219291 | |
Source | EPA DSSTox | |
URL | https://comptox.epa.gov/dashboard/DTXSID60585141 | |
Description | DSSTox provides a high quality public chemistry resource for supporting improved predictive toxicology. | |
Molecular Weight |
935.3 g/mol | |
Source | PubChem | |
URL | https://pubchem.ncbi.nlm.nih.gov | |
Description | Data deposited in or computed by PubChem | |
CAS No. |
107148-01-6 | |
Record name | PUBCHEM_16219291 | |
Source | EPA DSSTox | |
URL | https://comptox.epa.gov/dashboard/DTXSID60585141 | |
Description | DSSTox provides a high quality public chemistry resource for supporting improved predictive toxicology. | |
Disclaimer and Information on In-Vitro Research Products
Please be aware that all articles and product information presented on BenchChem are intended solely for informational purposes. The products available for purchase on BenchChem are specifically designed for in-vitro studies, which are conducted outside of living organisms. In-vitro studies, derived from the Latin term "in glass," involve experiments performed in controlled laboratory settings using cells or tissues. It is important to note that these products are not categorized as medicines or drugs, and they have not received approval from the FDA for the prevention, treatment, or cure of any medical condition, ailment, or disease. We must emphasize that any form of bodily introduction of these products into humans or animals is strictly prohibited by law. It is essential to adhere to these guidelines to ensure compliance with legal and ethical standards in research and experimentation.