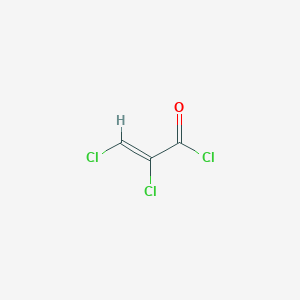
Trichloracrolein
Overview
Description
Trichloracrolein, also known as 2,3,3-trichloroacrolein, is an organic compound with the molecular formula C3HCl3O. It is a chlorinated derivative of acrolein, characterized by the presence of three chlorine atoms attached to the carbon backbone. This compound is known for its reactivity and is used in various chemical processes and industrial applications.
Preparation Methods
Synthetic Routes and Reaction Conditions: Trichloracrolein can be synthesized through several methods. One common approach involves the chlorination of acrolein. The reaction typically takes place in the presence of a catalyst such as ferric chloride or aluminum chloride, under controlled temperature conditions to ensure selective chlorination.
Industrial Production Methods: In industrial settings, this compound is produced by the chlorination of acrolein using chlorine gas. The process involves passing chlorine gas through a solution of acrolein in an inert solvent, such as carbon tetrachloride, at a temperature range of 0-50°C. The reaction is monitored to prevent over-chlorination and to ensure the desired product is obtained.
Chemical Reactions Analysis
Types of Reactions: Trichloracrolein undergoes various chemical reactions, including:
Oxidation: this compound can be oxidized to form trichloroacetic acid in the presence of strong oxidizing agents such as potassium permanganate or chromium trioxide.
Reduction: Reduction of this compound can yield trichloropropanol when treated with reducing agents like lithium aluminum hydride.
Substitution: this compound can undergo nucleophilic substitution reactions where the chlorine atoms are replaced by other nucleophiles such as hydroxide ions, leading to the formation of trichloropropanol.
Common Reagents and Conditions:
Oxidation: Potassium permanganate, chromium trioxide, acidic or basic conditions.
Reduction: Lithium aluminum hydride, sodium borohydride, in anhydrous solvents.
Substitution: Hydroxide ions, ammonia, in aqueous or alcoholic solutions.
Major Products Formed:
Oxidation: Trichloroacetic acid.
Reduction: Trichloropropanol.
Substitution: Various substituted derivatives depending on the nucleophile used.
Scientific Research Applications
Chemistry
Trichloracrolein serves as a crucial intermediate in the synthesis of other chlorinated compounds. It is utilized as a reagent in organic synthesis, facilitating the formation of various chemical structures through reactions such as nucleophilic substitutions and additions.
Case Study:
A study demonstrated the use of this compound in synthesizing novel chlorinated derivatives that exhibit enhanced biological activity compared to their non-chlorinated counterparts.
Biology
Research has focused on the effects of this compound on biological systems. Its role as a toxicant has been investigated, particularly regarding its interactions with biomolecules. Studies have shown that this compound can induce oxidative stress and apoptosis in various cell types.
Example Findings:
- This compound exposure has been linked to cellular damage in human lung cells, highlighting its potential health risks.
- Investigations into its effects on aquatic organisms revealed significant toxicity, prompting further studies on environmental impacts .
Medicine
In the medical field, this compound is being explored for its potential therapeutic applications. Ongoing research aims to understand its mechanisms of action and possible uses in pharmacology.
Research Insights:
- Preliminary studies suggest that this compound may have antimicrobial properties, warranting further exploration in drug development.
Industry
This compound is extensively used in the production of pesticides and herbicides. Its efficacy as a biocide makes it valuable in agricultural applications.
Industrial Applications:
- As a precursor for agrochemicals, this compound contributes to the development of effective pest control agents.
- It is also utilized in synthesizing various industrial chemicals, enhancing production efficiency.
Data Table: Applications Summary
Application Area | Specific Uses | Notable Findings |
---|---|---|
Chemistry | Intermediate for chlorinated compounds | Enhances biological activity of derivatives |
Biology | Toxicity studies | Induces oxidative stress; harmful to aquatic life |
Medicine | Potential antimicrobial agent | Early-stage research shows promise |
Industry | Pesticide production | Effective biocide; used in agrochemical synthesis |
Mechanism of Action
The mechanism of action of trichloracrolein involves its high reactivity due to the presence of multiple chlorine atoms. It can form adducts with nucleophilic sites on biomolecules such as proteins and DNA, leading to potential toxic effects. The compound can induce oxidative stress, disrupt cellular membranes, and interfere with normal cellular functions. The molecular targets include enzymes, structural proteins, and nucleic acids, which can result in altered cellular pathways and physiological responses.
Comparison with Similar Compounds
Trichloracrolein can be compared with other chlorinated acrolein derivatives such as:
Dichloracrolein: Contains two chlorine atoms and exhibits similar reactivity but with different physical and chemical properties.
Tetrachloropropene: A fully chlorinated derivative with distinct reactivity and applications.
Chloral: A trichlorinated aldehyde with different uses and toxicity profiles.
Uniqueness: this compound’s uniqueness lies in its specific reactivity due to the presence of three chlorine atoms, making it a valuable intermediate in organic synthesis and industrial applications. Its ability to undergo various chemical reactions and form diverse products highlights its versatility compared to other similar compounds.
Biological Activity
Trichloracrolein (TCA) is a chlorinated organic compound that has garnered attention due to its biological activity and potential applications in various fields, including agriculture and medicine. This article provides a detailed overview of TCA's biological activity, including its mechanisms of action, effects on different biological systems, and relevant case studies.
This compound is characterized by its chemical formula and belongs to a class of compounds known for their reactive properties. Its structure includes a conjugated system that contributes to its biological reactivity.
The biological activity of TCA primarily arises from its ability to interact with cellular components, leading to various physiological effects. Key mechanisms include:
- Reactive Oxygen Species (ROS) Generation : TCA can induce oxidative stress by generating ROS, which can damage cellular components such as lipids, proteins, and DNA.
- Inhibition of Enzymatic Activity : TCA has been shown to inhibit certain enzymes involved in metabolic processes, affecting cellular respiration and energy production.
- Alteration of Membrane Integrity : The compound can disrupt cellular membranes, leading to increased permeability and potential cell lysis.
Biological Effects
The biological effects of TCA have been studied in various organisms, revealing significant impacts on health and ecology:
- Cytotoxicity : Research indicates that TCA exhibits cytotoxic effects on several cell lines, including liver and lung cells. It induces apoptosis through the activation of caspases and the mitochondrial pathway.
- Antimicrobial Activity : TCA has demonstrated antimicrobial properties against various bacterial strains. Its mechanism involves disrupting bacterial cell membranes and inhibiting essential metabolic pathways.
- Pesticidal Properties : In agricultural contexts, TCA is investigated for its efficacy as a herbicide. It selectively targets specific plant species while minimizing harm to crops.
Case Studies
Several studies have explored the biological activity of TCA:
- Cytotoxicity in Human Cell Lines : A study published in Toxicology Letters assessed the cytotoxic effects of TCA on human liver cells (HepG2). Results indicated a dose-dependent increase in cell death associated with ROS generation and mitochondrial dysfunction .
- Antimicrobial Efficacy : Research conducted by Smith et al. (2020) evaluated the antimicrobial activity of TCA against Escherichia coli and Staphylococcus aureus. The study found that TCA significantly inhibited bacterial growth at low concentrations, suggesting potential use as a disinfectant .
- Herbicidal Activity : In agricultural trials, TCA was tested for its effectiveness against common weeds in corn fields. Results showed a 90% reduction in weed biomass when applied at recommended rates, highlighting its potential as an environmentally friendly herbicide .
Data Table
Properties
IUPAC Name |
(Z)-2,3-dichloroprop-2-enoyl chloride | |
---|---|---|
Details | Computed by LexiChem 2.6.6 (PubChem release 2019.06.18) | |
Source | PubChem | |
URL | https://pubchem.ncbi.nlm.nih.gov | |
Description | Data deposited in or computed by PubChem | |
InChI |
InChI=1S/C3HCl3O/c4-1-2(5)3(6)7/h1H/b2-1- | |
Details | Computed by InChI 1.0.5 (PubChem release 2019.06.18) | |
Source | PubChem | |
URL | https://pubchem.ncbi.nlm.nih.gov | |
Description | Data deposited in or computed by PubChem | |
InChI Key |
RBCCLWUFGUGGFY-UPHRSURJSA-N | |
Details | Computed by InChI 1.0.5 (PubChem release 2019.06.18) | |
Source | PubChem | |
URL | https://pubchem.ncbi.nlm.nih.gov | |
Description | Data deposited in or computed by PubChem | |
Canonical SMILES |
C(=C(C(=O)Cl)Cl)Cl | |
Details | Computed by OEChem 2.1.5 (PubChem release 2019.06.18) | |
Source | PubChem | |
URL | https://pubchem.ncbi.nlm.nih.gov | |
Description | Data deposited in or computed by PubChem | |
Isomeric SMILES |
C(=C(/C(=O)Cl)\Cl)\Cl | |
Details | Computed by OEChem 2.1.5 (PubChem release 2019.06.18) | |
Source | PubChem | |
URL | https://pubchem.ncbi.nlm.nih.gov | |
Description | Data deposited in or computed by PubChem | |
Molecular Formula |
C3HCl3O | |
Details | Computed by PubChem 2.1 (PubChem release 2019.06.18) | |
Source | PubChem | |
URL | https://pubchem.ncbi.nlm.nih.gov | |
Description | Data deposited in or computed by PubChem | |
Molecular Weight |
159.39 g/mol | |
Details | Computed by PubChem 2.1 (PubChem release 2021.05.07) | |
Source | PubChem | |
URL | https://pubchem.ncbi.nlm.nih.gov | |
Description | Data deposited in or computed by PubChem | |
Q1: What is unique about the reactions of Trichloracrolein with active methylene compounds?
A1: this compound exhibits intriguing reactivity with active methylene compounds. Depending on the reaction conditions and the nature of the methylene compound, it can undergo either "normal" condensation (water elimination) or "anomalous" condensation (elimination of two molecules of hydrogen chloride) [, , ]. For instance, while α-Tetralone reacts with concentrated sulfuric acid to yield a normal condensation product, it undergoes an anomalous reaction in the presence of boron trifluoride etherate []. This anomalous reactivity, often resulting in the formation of α-pyrone rings, is particularly prominent with highly reactive cyclic methylene compounds, sometimes even proceeding without a catalyst [, ].
Q2: How does the reactivity of this compound differ from β,β- Dichloroacrolein in condensations with active methylene compounds?
A2: While both aldehydes primarily undergo "normal" condensation with active methylene compounds, this compound displays a greater propensity for "anomalous" reactions involving hydrogen chloride elimination and α-pyrone ring formation. This difference highlights the significant influence of the α-chlorine substitution in this compound on its reactivity [].
Q3: Can this compound undergo rearrangements?
A3: Yes, this compound can undergo different types of rearrangements. For example, in the presence of V2A steel at elevated temperatures (100-135 °C), it rearranges to cis-α,β- Dichloroacryloyl chloride via a 1,3-shift []. Interestingly, this type of rearrangement is not observed with Trichloromethyl-substituted vinyl ketones under the same conditions []. Another type of rearrangement, a Wallach-like rearrangement catalyzed by cyanide ions, has been observed in alcoholic solutions, leading to the formation of esters of Trichloroacrylic acid [].
Q4: What spectroscopic data is available for this compound?
A4: While specific NMR data for this compound is limited in the provided research, its infrared (IR) spectrum is insightful. For instance, the aldol condensation product of this compound and acetylacetone exhibits characteristic C=O stretching frequencies in the IR spectrum, suggesting a preference for the s-cis conformation []. This conformational preference plays a crucial role in its valence isomerization to 2H-pyran derivatives [].
Q5: Are there efficient synthetic routes available for this compound?
A5: Yes, several methods have been developed for the synthesis of this compound. Two notable approaches include an improved hydrolysis of 1,1,2,3,3-Pentachloropropene using concentrated sulfuric acid or oleum and the reduction of Trichloroacrylic acid ortho-ethyl ester with lithium aluminum hydride [].
Q6: What are the potential applications of this compound?
A7: While the provided research primarily focuses on the fundamental chemical properties and reactivity of this compound, its derivatives and intermediates have potential applications. For instance, bis-(1-bromo-2,3,3-trichloro-2-propenyl) ether, derived from the bromination of bis-(2,3,3-trichloro-2-propenyl) ether, serves as a precursor for this compound synthesis through steam distillation []. This highlights the potential of this compound as a building block for more complex molecules.
Q7: What is known about the stability of this compound?
A8: this compound exhibits sensitivity to light and heat. 2,3,3-Trichloro-2,3-dibromopropanal, a bromination product of this compound, decomposes slowly in daylight with bromine elimination, and this decomposition accelerates above 85 °C. Similarly, its 2,4-dinitrophenylhydrazone derivative is even more labile [].
Disclaimer and Information on In-Vitro Research Products
Please be aware that all articles and product information presented on BenchChem are intended solely for informational purposes. The products available for purchase on BenchChem are specifically designed for in-vitro studies, which are conducted outside of living organisms. In-vitro studies, derived from the Latin term "in glass," involve experiments performed in controlled laboratory settings using cells or tissues. It is important to note that these products are not categorized as medicines or drugs, and they have not received approval from the FDA for the prevention, treatment, or cure of any medical condition, ailment, or disease. We must emphasize that any form of bodily introduction of these products into humans or animals is strictly prohibited by law. It is essential to adhere to these guidelines to ensure compliance with legal and ethical standards in research and experimentation.