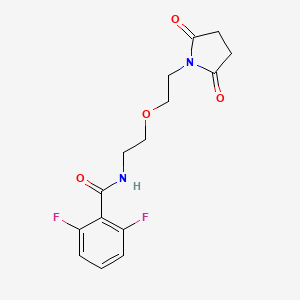
N-(2-(2-(2,5-dioxopyrrolidin-1-yl)ethoxy)ethyl)-2,6-difluorobenzamide
Overview
Description
N-(2-(2-(2,5-dioxopyrrolidin-1-yl)ethoxy)ethyl)-2,6-difluorobenzamide is a synthetic organic compound characterized by a 2,6-difluorobenzamide core linked via a polyethylene glycol (PEG)-like ethoxyethyl chain to a 2,5-dioxopyrrolidin-1-yl group. The 2,5-dioxopyrrolidin moiety functions as an activated ester, akin to N-hydroxysuccinimide (NHS), enabling efficient conjugation with primary amines under mild conditions . The synthesis of related compounds involves carbodiimide-mediated coupling (e.g., DCC) to activate carboxyl groups, followed by NHS ester formation for stability and reactivity .
The ethoxyethyl spacer improves solubility and reduces steric hindrance during conjugation.
Preparation Methods
Synthetic Routes and Reaction Conditions
The synthesis of N-(2-(2-(2,5-dioxopyrrolidin-1-yl)ethoxy)ethyl)-2,6-difluorobenzamide typically involves a multi-step process:
Formation of the Pyrrolidinone Intermediate: The initial step involves the preparation of 2,5-dioxopyrrolidin-1-yl, which can be synthesized through the reaction of succinic anhydride with ammonia or an amine under controlled conditions.
Ethoxylation: The pyrrolidinone intermediate is then reacted with ethylene oxide to introduce the ethoxy groups. This step requires careful control of temperature and pressure to ensure the desired substitution.
Coupling with Difluorobenzamide: The final step involves coupling the ethoxylated pyrrolidinone with 2,6-difluorobenzoyl chloride in the presence of a base such as triethylamine. This reaction is typically carried out in an inert atmosphere to prevent unwanted side reactions.
Industrial Production Methods
Industrial production of this compound may involve similar synthetic routes but on a larger scale, with optimizations for yield and purity. Techniques such as continuous flow synthesis and the use of automated reactors can enhance efficiency and scalability.
Chemical Reactions Analysis
Types of Reactions
N-(2-(2-(2,5-dioxopyrrolidin-1-yl)ethoxy)ethyl)-2,6-difluorobenzamide can undergo various chemical reactions, including:
Substitution Reactions: The compound can participate in nucleophilic substitution reactions, particularly at the benzamide moiety.
Oxidation and Reduction: The pyrrolidinone ring can be subjected to oxidation or reduction, altering its chemical properties.
Hydrolysis: The ester linkages in the compound can be hydrolyzed under acidic or basic conditions, leading to the formation of corresponding acids and alcohols.
Common Reagents and Conditions
Nucleophilic Substitution: Reagents such as sodium hydride or potassium carbonate in polar aprotic solvents like dimethylformamide (DMF) are commonly used.
Oxidation: Oxidizing agents like potassium permanganate or hydrogen peroxide can be employed.
Reduction: Reducing agents such as lithium aluminum hydride (LiAlH4) or sodium borohydride (NaBH4) are effective.
Hydrolysis: Acidic conditions (e.g., hydrochloric acid) or basic conditions (e.g., sodium hydroxide) can be used for hydrolysis.
Major Products
The major products formed from these reactions depend on the specific conditions and reagents used. For example, hydrolysis of the compound can yield 2,6-difluorobenzoic acid and the corresponding ethoxyethyl alcohol.
Scientific Research Applications
Antimicrobial Activity
Research indicates that derivatives of 2,6-difluorobenzamide exhibit significant antimicrobial properties. In particular, studies have focused on the efficacy of fluorinated benzamide derivatives against various bacterial strains, including Staphylococcus aureus. The incorporation of fluorine atoms in the structure enhances the conformational stability of these compounds, leading to improved interactions with bacterial targets .
Central Nervous System Disorders
N-(2-(2-(2,5-dioxopyrrolidin-1-yl)ethoxy)ethyl)-2,6-difluorobenzamide has been investigated for its potential as a modulator of excitatory amino acid transporters (EAATs), which play a crucial role in neurotransmission and are implicated in conditions such as epilepsy. The compound has shown promising pharmacokinetic profiles and may serve as a first-in-class small-molecule positive allosteric modulator for EAAT2 .
Application | Mechanism | Potential Condition | Reference |
---|---|---|---|
EAAT Modulation | Positive Allosteric Modulator | Epilepsy |
Drug Design and Development
The unique structure of this compound allows for the exploration of new drug candidates with enhanced bioavailability and efficacy. Molecular docking studies suggest that the compound can effectively bind to specific protein targets involved in disease pathways, making it a candidate for further development in therapeutic applications .
Case Study: Fluorinated Benzamides in Drug Discovery
A recent study analyzed the binding affinities of various fluorinated benzamides to their target proteins. The results indicated that compounds with the 2,6-difluoro substitution exhibited higher potency compared to non-fluorinated analogs due to improved molecular interactions .
Polymer Chemistry
The compound's structural features have also found applications in polymer chemistry. It can be used as a building block for synthesizing novel polymers with tailored properties for specific applications such as drug delivery systems or biodegradable materials.
Mechanism of Action
The mechanism by which N-(2-(2-(2,5-dioxopyrrolidin-1-yl)ethoxy)ethyl)-2,6-difluorobenzamide exerts its effects depends on its specific application. In medicinal chemistry, it may act by binding to a target enzyme or receptor, inhibiting its activity. The molecular targets and pathways involved can vary, but typically include interactions with proteins or nucleic acids, leading to modulation of biological processes.
Comparison with Similar Compounds
Comparison with Structurally Similar Compounds
The compound shares structural motifs with benzamide derivatives, particularly agrochemicals and biochemical tools. Below is a comparative analysis:
Structural and Functional Group Analysis
Key Differences and Implications
Substituent Positioning: The 2,6-difluoro substitution on the benzamide ring distinguishes the target compound from herbicides like etobenzanid (2,3-dichloro) and diflufenican (2,4-difluoro).
Functional Groups :
- The 2,5-dioxopyrrolidin group in the target compound contrasts with the ethoxymethoxy (etobenzanid) or pyridinecarboxamide (diflufenican) moieties. This reactive ester enables covalent bonding with amines, unlike the stable ether or carboxamide groups in pesticides.
Solubility and Reactivity: The ethoxyethyl chain in the target compound enhances hydrophilicity compared to the lipophilic trifluoromethylphenoxy group in diflufenican. This improves compatibility with aqueous systems, critical for biochemical applications.
Mechanistic Divergence :
- While etobenzanid and diflufenican inhibit plant-specific enzymes (e.g., phytoene desaturase), the target compound’s NHS ester suggests utility in crosslinking or labeling biomolecules, aligning with synthetic strategies in .
Biological Activity
N-(2-(2-(2,5-dioxopyrrolidin-1-yl)ethoxy)ethyl)-2,6-difluorobenzamide is a synthetic compound that has garnered attention for its potential biological activities, particularly in the fields of pharmacology and medicinal chemistry. This article explores the compound's biological activity, mechanisms of action, and relevant research findings.
Chemical Structure and Properties
- Molecular Formula : C15H20F2N2O3
- Molecular Weight : 320.33 g/mol
- CAS Number : 2034537-89-6
The compound features a pyrrolidine ring, a difluorobenzamide moiety, and an ethoxyethyl side chain, which contribute to its biological properties.
Research indicates that compounds with similar structures exhibit various pharmacological effects, including:
- Anticonvulsant Activity : The compound may inhibit calcium currents mediated by L-type calcium channels. This inhibition is crucial in reducing neuronal excitability, making it a candidate for anticonvulsant therapies .
- FtsZ Inhibition : The 2,6-difluorobenzamide component suggests potential activity against bacterial cell division through inhibition of the FtsZ protein, which is essential for bacterial cytokinesis .
Anticonvulsant Properties
A study focusing on hybrid compounds containing the 2,5-dioxopyrrolidin-1-yl moiety demonstrated significant anticonvulsant effects in various animal models. The compound was tested using:
- Maximal Electroshock (MES) Test
- Pentylenetetrazole (PTZ) Test
Results indicated that compounds derived from similar frameworks provided protection against seizures with favorable safety profiles compared to established antiepileptic drugs like valproic acid .
Pharmacokinetics and ADME-Tox Profile
The pharmacokinetic properties of this compound suggest:
- High Metabolic Stability : The compound exhibited good stability in human liver microsomes.
- Permeability : It demonstrated favorable permeability characteristics in membrane permeability assays.
- Cytochrome P450 Interaction : Moderate inhibition of CYP2C9 was noted without significant effects on CYP3A4 or CYP2D6 activities .
Case Studies and Research Findings
Q & A
Q. Basic: What synthetic strategies are employed for introducing the 2,5-dioxopyrrolidin-1-yl (NHS ester) group into benzamide derivatives, and how do reaction conditions influence yield?
Methodological Answer:
The 2,5-dioxopyrrolidin-1-yl (NHS ester) group is typically introduced via carbodiimide-mediated coupling. For example, a derivative with a similar PEG-like spacer was synthesized by reacting a precursor with N-hydroxysuccinimide (NHS) and dicyclohexylcarbodiimide (DCC) in dichloromethane (DCM) under overnight stirring . Key considerations:
- Reagent Ratios: Excess NHS (4.4 equiv) and DCC (2.5 equiv) ensure complete activation of carboxylic acid intermediates.
- Solvent Choice: DCM is preferred for its low polarity, which minimizes side reactions.
- Purification: Evaporation under reduced pressure followed by column chromatography removes unreacted reagents.
Yield optimization requires strict anhydrous conditions and monitoring via TLC or LC-MS to detect intermediates.
Q. Basic: How can researchers confirm the successful conjugation of the PEG-like spacer in this compound using spectroscopic techniques?
Methodological Answer:
- ¹H/¹³C NMR: Characteristic peaks for the PEG-like spacer (e.g., ethylene oxide protons at δ 3.5–3.7 ppm) and the NHS ester (carbonyl carbons at ~170 ppm) should appear. Disappearance of the precursor’s carboxylic acid proton (δ ~12 ppm) confirms conjugation .
- IR Spectroscopy: A strong C=O stretch (~1640–1695 cm⁻¹) for the amide bond and the absence of a free carboxylic acid O–H stretch (~2500–3300 cm⁻¹) validate successful coupling .
- Mass Spectrometry: High-resolution MS (HRMS) or MALDI-TOF can confirm the molecular ion peak matching the expected mass.
Q. Advanced: What analytical challenges arise in resolving crystallographic disorder in difluorobenzamide derivatives, and how can they be addressed?
Methodological Answer:
Crystallographic disorder, as observed in structurally similar compounds, arises from flexible substituents (e.g., ethoxy chains) or rotational freedom. Strategies include:
- Low-Temperature Data Collection: Reduces thermal motion, improving electron density maps.
- Multi-Conformer Modeling: Refining alternative positions for disordered atoms with occupancy constraints.
- Hydrogen Bond Analysis: Identifying supramolecular interactions (e.g., N–H⋯N dimers) to anchor molecular orientation .
For example, a study on 2,3-difluoro-N-(2-pyridyl)benzamide resolved disorder by analyzing hydrogen-bonded dimers and using restraints during refinement .
Q. Advanced: How does the electronic environment of the difluorobenzamide core influence hydrogen-bonding patterns in supramolecular assemblies?
Methodological Answer:
The electron-withdrawing fluorine atoms polarize the benzamide ring, enhancing hydrogen-bond acceptor strength at the carbonyl oxygen. In 2,3-difluoro-N-(2-pyridyl)benzamide, this polarization stabilizes N–H⋯N interactions between amide protons and pyridyl nitrogen atoms, forming R₂²(8) dimer motifs . Computational studies (e.g., electrostatic potential maps) can quantify this effect. Additionally, steric hindrance from fluorine substituents may limit conformational flexibility, directing specific packing modes.
Q. Basic: What purification techniques are recommended for isolating N-(2-(2-(2,5-dioxopyrrolidin-1-yl)ethoxy)ethyl)-2,6-difluorobenzamide from reaction mixtures?
Methodological Answer:
- Liquid-Liquid Extraction: Use DCM/water to remove hydrophilic byproducts (e.g., dicyclohexylurea from DCC reactions) .
- Column Chromatography: Silica gel with gradient elution (e.g., hexane/ethyl acetate) separates the product from unreacted NHS ester or PEG-like intermediates.
- Recrystallization: Ethanol or acetone can yield high-purity crystals, monitored by melting point analysis (e.g., 348–352 K for related compounds) .
Q. Advanced: How can researchers design analogs of this compound to enhance stability in aqueous biological systems?
Methodological Answer:
- Spacer Modification: Replacing the ethylene glycol chain with polypropylene glycol reduces hydrophilicity, slowing hydrolysis.
- Prodrug Strategies: Masking the NHS ester as a stable precursor (e.g., tert-butyl carbamate) that activates under specific pH or enzymatic conditions .
- Conjugation with Polymers: Incorporating the benzamide into coordination polymers or PEGylated systems (e.g., PT-ADA-PPR in biomedical materials) improves solubility and stability .
Q. Basic: What spectroscopic or chromatographic methods are critical for quantifying residual solvents or unreacted reagents in the final product?
Methodological Answer:
- GC-MS: Quantifies volatile residuals (e.g., DCM, ethyl acetate) with detection limits <1 ppm.
- ¹⁹F NMR: Detects fluorinated impurities (e.g., unreacted 2,6-difluorobenzoyl chloride) due to distinct chemical shifts.
- HPLC with UV Detection: Uses a C18 column and acetonitrile/water gradient to separate and quantify NHS ester byproducts.
Q. Advanced: What mechanistic insights guide the optimization of coupling reactions involving NHS esters in similar benzamide derivatives?
Methodological Answer:
- Reaction Kinetics: NHS esters hydrolyze in aqueous environments; thus, reactions must proceed in anhydrous solvents with molecular sieves.
- Catalyst Selection: DMAP (4-dimethylaminopyridine) accelerates acyl transfer in sterically hindered systems.
- Byproduct Management: Dicyclohexylurea precipitates in DCM, simplifying removal. Studies on fullerene-malonate conjugates highlight the importance of stoichiometric excess and reaction time (~12–24 hours) .
Q. Basic: How can researchers validate the hydrolytic stability of the NHS ester group under physiological conditions?
Methodological Answer:
- pH-Dependent Studies: Incubate the compound in buffers (pH 4.0–7.4) and monitor hydrolysis via HPLC or ¹H NMR.
- Mass Loss Analysis: Track degradation products (e.g., free carboxylic acid) over time.
- Comparative Studies: Use analogs with stabilized esters (e.g., sulfonated NHS) as controls.
Q. Advanced: What structural features of this compound make it suitable for targeted drug delivery systems?
Methodological Answer:
- PEG-like Spacer: Enhances solubility and prolongs circulation time in vivo.
- NHS Ester Reactivity: Enables covalent conjugation to amine-rich biomolecules (e.g., lysine residues in proteins) .
- Fluorine Substituents: Improves membrane permeability and bioavailability. Studies on similar difluorobenzamides demonstrate applications in antiviral and anticancer therapies .
Properties
IUPAC Name |
N-[2-[2-(2,5-dioxopyrrolidin-1-yl)ethoxy]ethyl]-2,6-difluorobenzamide | |
---|---|---|
Details | Computed by Lexichem TK 2.7.0 (PubChem release 2021.05.07) | |
Source | PubChem | |
URL | https://pubchem.ncbi.nlm.nih.gov | |
Description | Data deposited in or computed by PubChem | |
InChI |
InChI=1S/C15H16F2N2O4/c16-10-2-1-3-11(17)14(10)15(22)18-6-8-23-9-7-19-12(20)4-5-13(19)21/h1-3H,4-9H2,(H,18,22) | |
Details | Computed by InChI 1.0.6 (PubChem release 2021.05.07) | |
Source | PubChem | |
URL | https://pubchem.ncbi.nlm.nih.gov | |
Description | Data deposited in or computed by PubChem | |
InChI Key |
YHYVTXBPMNGZEP-UHFFFAOYSA-N | |
Details | Computed by InChI 1.0.6 (PubChem release 2021.05.07) | |
Source | PubChem | |
URL | https://pubchem.ncbi.nlm.nih.gov | |
Description | Data deposited in or computed by PubChem | |
Canonical SMILES |
C1CC(=O)N(C1=O)CCOCCNC(=O)C2=C(C=CC=C2F)F | |
Details | Computed by OEChem 2.3.0 (PubChem release 2021.05.07) | |
Source | PubChem | |
URL | https://pubchem.ncbi.nlm.nih.gov | |
Description | Data deposited in or computed by PubChem | |
Molecular Formula |
C15H16F2N2O4 | |
Details | Computed by PubChem 2.1 (PubChem release 2021.05.07) | |
Source | PubChem | |
URL | https://pubchem.ncbi.nlm.nih.gov | |
Description | Data deposited in or computed by PubChem | |
Molecular Weight |
326.29 g/mol | |
Details | Computed by PubChem 2.1 (PubChem release 2021.05.07) | |
Source | PubChem | |
URL | https://pubchem.ncbi.nlm.nih.gov | |
Description | Data deposited in or computed by PubChem | |
Disclaimer and Information on In-Vitro Research Products
Please be aware that all articles and product information presented on BenchChem are intended solely for informational purposes. The products available for purchase on BenchChem are specifically designed for in-vitro studies, which are conducted outside of living organisms. In-vitro studies, derived from the Latin term "in glass," involve experiments performed in controlled laboratory settings using cells or tissues. It is important to note that these products are not categorized as medicines or drugs, and they have not received approval from the FDA for the prevention, treatment, or cure of any medical condition, ailment, or disease. We must emphasize that any form of bodily introduction of these products into humans or animals is strictly prohibited by law. It is essential to adhere to these guidelines to ensure compliance with legal and ethical standards in research and experimentation.