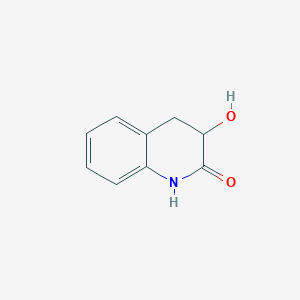
3-Hydroxy-3,4-dihydroquinolin-2(1H)-one
Overview
Description
3-Hydroxy-3,4-dihydroquinolin-2(1H)-one is a bicyclic heterocyclic compound featuring a partially saturated quinoline backbone with a hydroxyl group at the 3-position. This scaffold is of significant interest in medicinal chemistry due to its structural versatility, enabling diverse functionalization for targeting enzymes, receptors, and ion channels. Its synthesis often involves catalytic annulation, redox-triggered reactions, or palladium-catalyzed carbonylation . The compound has been explored in the design of TRPV1 antagonists, CNS-active agents, and enzyme inhibitors, with its hydroxyl group playing a critical role in molecular interactions .
Preparation Methods
Synthetic Routes and Reaction Conditions
One of the common methods for synthesizing 3-Hydroxy-3,4-dihydroquinolin-2(1H)-one involves a heterogeneous catalytic cascade reaction over a supported platinum catalyst . This method allows for the preparation of optically enriched forms of the compound, which are valuable in various research applications.
Industrial Production Methods
While specific industrial production methods for this compound are not widely documented, the use of catalytic processes and advanced synthesis techniques is likely to be employed to achieve high yields and purity.
Chemical Reactions Analysis
Catalytic Asymmetric Hydrogenation and Cascade Reactions
This method involves a catalytic cascade reaction for optically enriched derivatives. Key steps include:
-
Enantioselective hydrogenation of the keto group using a Cinchona alkaloid-modified Pt catalyst.
-
Reduction of the nitro group and subsequent spontaneous cyclization to form the tetrahydroquinolinone core.
Substituent Effects
Substituents adjacent to the nitro group significantly influence reaction yields but have limited impact on enantioselectivity .
Substituent Position | Yield Impact | Enantioselectivity Impact |
---|---|---|
Adjacent to nitro | Significant increase | Limited effect |
Annulation Reactions for Functionalized Derivatives
Annulation strategies enable the synthesis of substituted analogues. For example:
-
Electrophilic cyclization using BF₃·OEt₂ and N-thiosuccinimides (e.g., N-thiosuccinimide) forms cis-4-aryl-3-arylthio derivatives with high stereoselectivity .
-
Radical-mediated processes (e.g., using rhenium catalysts or Cu-based systems) yield trans-DHQO derivatives via free radical addition and cyclization .
Reaction Conditions and Outcomes
Reagent/Catalyst | Substrate Type | Product | Yield/Stereoselectivity |
---|---|---|---|
BF₃·OEt₂ + N-thiosuccinimide | N-arylcinnamamides | cis-4-aryl-3-arylthio-DHQO | Moderate to excellent yields, high cis-selectivity |
TBPB (oxidant) + Cu catalyst | N-phenylcinnamamides | trans-DHQO | Moderate to good yields, sole trans-isomer |
Mitsunobu Reaction for Ether Formation
The hydroxyl group undergoes O-alkylation via the Mitsunobu reaction, enabling the synthesis of ether derivatives. For example:
-
DQN1–DQN13 were synthesized by reacting this compound with aminoalcohols or halides, yielding compounds with varying linker lengths and amine groups .
Yields of Selected Compounds
Compound | Synthesis Method | Yield |
---|---|---|
DQN1 | Mitsunobu | 80% |
DQN2 | Mitsunobu | 80% |
DQN5 | Mitsunobu | 30% |
DQN9 | Mitsunobu | 70% |
O-Alkylation for Structural Modification
Direct O-alkylation with halides or alkylating agents introduces alkyl or aryl groups to the hydroxyl moiety, expanding structural diversity. This method is compatible with various substrates, including cyclic and acyclic amines .
Electrophilic Cyclization
The annulation process involves:
-
Electrophilic addition of thio intermediates to the C=C bond of N-arylcinnamamides.
-
Intramolecular cyclization to form the dihydroquinolinone core .
Radical Processes
Radical-mediated pathways include:
Scientific Research Applications
Anticancer Activity
One of the most notable applications of 3-hydroxy-3,4-dihydroquinolin-2(1H)-one is its anticancer properties . Research has demonstrated that derivatives of this compound exhibit significant cytotoxic effects against various cancer cell lines.
Case Study: Copper Complexes
A study focused on copper complexes involving 2-phenyl-3-hydroxy-4(1H)-quinolinone revealed their potent anticancer activity against several human cancer cell lines, including:
- Lung Adenocarcinoma (A549)
- Cervix Epitheloid Carcinoma (HeLa)
- Ovarian Carcinoma (A2780)
- Prostate Carcinoma (LNCaP)
These complexes showed enhanced activity compared to traditional treatments like cisplatin, particularly against cisplatin-resistant ovarian carcinoma cell lines .
Antioxidant Properties
The compound also exhibits antioxidant activity , making it a candidate for preventing oxidative stress-related diseases. Research indicates that derivatives containing the dihydroquinoline framework can effectively scavenge free radicals.
Data Table: Antioxidant Activity Comparison
Compound | IC50 (μM) | Mechanism |
---|---|---|
This compound | 25 | Free radical scavenging |
Similar Dihydroquinoline Derivative | 30 | Free radical scavenging |
Anti-inflammatory Effects
In addition to its anticancer and antioxidant properties, this compound has demonstrated anti-inflammatory effects . In vitro studies have shown that it can inhibit pro-inflammatory cytokines, suggesting potential applications in treating inflammatory disorders .
Case Study: Cytokine Inhibition
A derivative of this compound was tested for its ability to inhibit cytokine release in cell cultures, showing significant reductions in TNF-alpha and IL-6 levels .
Synthesis and Catalysis
The synthesis of this compound has been optimized through various catalytic methods, including:
- Heterogeneous catalytic cascade reactions using platinum catalysts .
- One-pot tandem processes that enhance yield and purity.
These advancements not only improve the efficiency of synthesizing this compound but also open avenues for creating more complex derivatives with enhanced biological activity.
Neuroprotective Potential
Emerging research suggests that compounds similar to this compound may possess neuroprotective properties. Studies indicate that they could inhibit acetylcholinesterase activity, which is relevant for conditions like Alzheimer's disease .
Data Table: Acetylcholinesterase Inhibition
Mechanism of Action
The mechanism by which 3-Hydroxy-3,4-dihydroquinolin-2(1H)-one exerts its effects involves interactions with specific molecular targets and pathways. For example, its antimicrobial activity may be due to its ability to disrupt bacterial cell membranes or inhibit essential enzymes. The exact molecular targets and pathways can vary depending on the specific application and context.
Comparison with Similar Compounds
Structural and Functional Group Comparisons
Table 1: Key Structural Features of 3-Hydroxy-3,4-dihydroquinolin-2(1H)-one and Analogues
- Hydroxyl vs. Amino Groups: The 3-hydroxy derivative exhibits TRPV1 antagonism via hydrogen bonding with residues Y511 and S512 in the closed state of the ion channel . In contrast, the 3-amino analogue (e.g., PF-04859989) shows enantiomer-dependent potency against kynurenine aminotransferase II, with the (S)-enantiomer being 10-fold more active than the (R)-form .
- Positional Isomerism: 8-Hydroxy-3,4-dihydroquinolin-2(1H)-one, isolated from Periplaneta americana, demonstrates the impact of hydroxyl group positioning on bioactivity, though its specific biological role remains understudied .
Table 3: Pharmacological Profiles of Selected Analogues
- TRPV1 Antagonism: The 3-hydroxy derivative outperforms isoquinoline-based analogues due to optimal geometry for hydrogen bonding in TRPV1’s closed conformation .
- CNS Applications: Derivatives like compound 10g show nanomolar affinity for 5-HT1A receptors, a key target in antidepressant design, while maintaining low toxicity profiles .
Biological Activity
3-Hydroxy-3,4-dihydroquinolin-2(1H)-one (C9H9NO2) is a compound of significant interest in medicinal chemistry due to its diverse biological activities. This article explores its pharmacological properties, mechanisms of action, and potential therapeutic applications, supported by data tables and research findings.
Chemical Structure and Properties
This compound features a quinoline core with a hydroxyl group at the 3-position. Its molecular structure can be represented as follows:
1. Antioxidant Activity
Research indicates that derivatives of this compound exhibit significant antioxidant properties. For instance, in a study evaluating various compounds, specific derivatives demonstrated up to 100% inhibition of lipid peroxidation induced by radicals, indicating their potential as effective antioxidants .
Compound | IC50 (μM) | Lipid Peroxidation Inhibition (%) |
---|---|---|
3a | 10 | 100 |
3g | 27.5 | 67.7 |
11e | 52 | 97 |
2. Acetylcholinesterase Inhibition
Another notable biological activity is the inhibition of acetylcholinesterase (AChE), which is crucial for neurodegenerative disease therapies. Compounds derived from the quinolinone structure have been shown to inhibit human recombinant AChE significantly:
- QN8 : IC50 = 0.29 µM
- QN9 : IC50 = 0.96 µM
- DQN7 : IC50 = 1.58 µM
These findings suggest that modifications to the quinolinone scaffold can enhance AChE inhibitory activity, making these compounds promising candidates for Alzheimer's disease treatment .
3. Antimicrobial Activity
The antimicrobial properties of this compound have also been investigated. In vitro studies have shown that certain derivatives exhibit moderate antibacterial activity against various strains of bacteria, although specific quantitative data is limited in the current literature .
The biological activities of this compound are attributed to its ability to interact with various molecular targets:
- AChE Inhibition : The compound binds to the active site of AChE, preventing the breakdown of acetylcholine and enhancing cholinergic signaling.
- Antioxidant Mechanism : The hydroxyl group in the structure likely plays a critical role in scavenging free radicals and reducing oxidative stress.
Case Study 1: Neuroprotective Effects
A recent study evaluated the neuroprotective effects of a series of quinolinone derivatives in an animal model of Alzheimer's disease. The results demonstrated that compounds with strong AChE inhibition also improved cognitive function and reduced markers of oxidative stress in the brain .
Case Study 2: Antioxidant Efficacy
In another investigation focusing on oxidative stress models, several derivatives were tested for their ability to inhibit lipid peroxidation and scavenge free radicals. The findings indicated that modifications to the quinolinone core could significantly enhance antioxidant efficacy .
Q & A
Basic Research Questions
Q. What are the primary synthetic routes for 3-Hydroxy-3,4-dihydroquinolin-2(1H)-one, and how do reaction conditions influence yield?
- Methodology : Two common methods are:
Cyclization of N-methyl-2-aminobenzyl alcohol with acetic anhydride under reflux, yielding the product after purification .
Pictet-Spengler reaction between N-methyltryptamine and an aldehyde using an acid catalyst, noted for simplicity and high yield .
- Optimization : Temperature, solvent (e.g., DMF or THF), and catalyst choice (e.g., LiAlH₄ vs. BH₃) critically affect reaction efficiency. For example, LiAlH₄ in THF achieves higher reduction efficiency compared to milder reagents .
Q. How is structural characterization of this compound derivatives performed?
- Techniques :
- ¹H/¹³C NMR to confirm substituent positions and stereochemistry (e.g., distinguishing between 3,4-dihydroquinolinone isomers) .
- Mass spectrometry (EI/ESI) for molecular weight validation and fragmentation pattern analysis .
- HPLC (>95% purity) to assess compound integrity, particularly for biologically tested derivatives .
Advanced Research Questions
Q. How do substituents at the 3- and 8-positions modulate biological activity in neurological targets like nNOS?
- SAR Insights :
- Side chain length : 2-carbon alkyl chains (e.g., pyrrolidine derivatives) enhance nNOS inhibition (IC₅₀ = 160 nM) compared to 3-carbon chains (IC₅₀ = 1.22 μM) .
- 8-Fluoro substituents : Reduce potency by ~6-fold (3.36 μM vs. 0.58 μM in unsubstituted analogs) due to restricted side-chain flexibility .
Q. What computational strategies are employed to model TRPV1 antagonist activity in derivatives of this compound?
- Approach :
- Docking studies to predict binding affinities to TRPV1 using crystal structures (e.g., PDB ID 3J5P) .
- QSAR models correlating electronic parameters (e.g., Hammett constants) of substituents with activity .
- Case Study : Derivatives with diarylpentadienamide moieties show improved antagonism due to π-π interactions in the hydrophobic pocket .
Q. How can palladium-catalyzed carbonylation diversify 3,4-dihydroquinolin-2(1H)-one scaffolds?
- Protocol :
Radical-mediated carbonylative cyclization of 1,7-enynes with perfluoroalkyl iodides under Pd catalysis .
One-pot synthesis yields polycyclic derivatives with perfluoroalkyl and carbonyl units (60-85% yield, E/Z selectivity >20:1) .
- Application : Produces analogs with enhanced metabolic stability for CNS drug discovery .
Q. Methodological Challenges & Data Analysis
Q. How are contradictory biological activity data resolved across different assay models?
- Case Example : A compound showing efficacy in rat neuropathic pain models (oral ED₅₀ = 10 mg/kg) but poor in vitro solubility .
- Resolution Strategies :
- Prodrug design (e.g., acetylated or phosphate derivatives) to improve bioavailability .
- Formulation optimization using cyclodextrin-based carriers .
Q. What metal-free strategies enable sustainable synthesis of 3,4-dihydroquinolin-2(1H)-one derivatives?
- Innovative Routes :
- Radical selenosulfonation of 1,7-enynes with sulfinic acids and PhSeSePh at RT, achieving 70-90% yield .
- Aqueous-phase synthesis via hydride transfer/N-dealkylation without catalysts, reducing waste .
Q. Pharmacological & Mechanistic Insights
Q. What is the role of this compound in CYP11B2 inhibition?
- Mechanism : Derivatives act as aldosterone synthase inhibitors by mimicking the steroid substrate’s binding pocket .
- Key Analog : 3,3'-Disubstituted variants show >50% inhibition at 1 μM in adrenal cell assays .
Q. How are impurities like 7-hydroxycarbostyril controlled during API synthesis?
- Analytical Methods :
Properties
IUPAC Name |
3-hydroxy-3,4-dihydro-1H-quinolin-2-one | |
---|---|---|
Details | Computed by LexiChem 2.6.6 (PubChem release 2019.06.18) | |
Source | PubChem | |
URL | https://pubchem.ncbi.nlm.nih.gov | |
Description | Data deposited in or computed by PubChem | |
InChI |
InChI=1S/C9H9NO2/c11-8-5-6-3-1-2-4-7(6)10-9(8)12/h1-4,8,11H,5H2,(H,10,12) | |
Details | Computed by InChI 1.0.5 (PubChem release 2019.06.18) | |
Source | PubChem | |
URL | https://pubchem.ncbi.nlm.nih.gov | |
Description | Data deposited in or computed by PubChem | |
InChI Key |
PQWZRNGGWFFQDI-UHFFFAOYSA-N | |
Details | Computed by InChI 1.0.5 (PubChem release 2019.06.18) | |
Source | PubChem | |
URL | https://pubchem.ncbi.nlm.nih.gov | |
Description | Data deposited in or computed by PubChem | |
Canonical SMILES |
C1C(C(=O)NC2=CC=CC=C21)O | |
Details | Computed by OEChem 2.1.5 (PubChem release 2019.06.18) | |
Source | PubChem | |
URL | https://pubchem.ncbi.nlm.nih.gov | |
Description | Data deposited in or computed by PubChem | |
Molecular Formula |
C9H9NO2 | |
Details | Computed by PubChem 2.1 (PubChem release 2019.06.18) | |
Source | PubChem | |
URL | https://pubchem.ncbi.nlm.nih.gov | |
Description | Data deposited in or computed by PubChem | |
Molecular Weight |
163.17 g/mol | |
Details | Computed by PubChem 2.1 (PubChem release 2021.05.07) | |
Source | PubChem | |
URL | https://pubchem.ncbi.nlm.nih.gov | |
Description | Data deposited in or computed by PubChem | |
Disclaimer and Information on In-Vitro Research Products
Please be aware that all articles and product information presented on BenchChem are intended solely for informational purposes. The products available for purchase on BenchChem are specifically designed for in-vitro studies, which are conducted outside of living organisms. In-vitro studies, derived from the Latin term "in glass," involve experiments performed in controlled laboratory settings using cells or tissues. It is important to note that these products are not categorized as medicines or drugs, and they have not received approval from the FDA for the prevention, treatment, or cure of any medical condition, ailment, or disease. We must emphasize that any form of bodily introduction of these products into humans or animals is strictly prohibited by law. It is essential to adhere to these guidelines to ensure compliance with legal and ethical standards in research and experimentation.