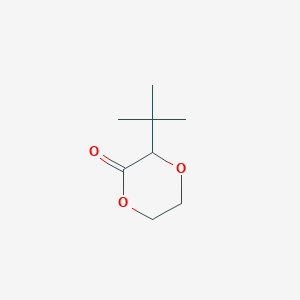
3-Tert-butyl-1,4-dioxan-2-one
- Click on QUICK INQUIRY to receive a quote from our team of experts.
- With the quality product at a COMPETITIVE price, you can focus more on your research.
Overview
Description
3-Tert-butyl-1,4-dioxan-2-one is an organic compound with the molecular formula C8H14O3 It is a cyclic carbonate with a tert-butyl group attached to the third carbon atom of the dioxane ring
Preparation Methods
Synthetic Routes and Reaction Conditions
The synthesis of 3-Tert-butyl-1,4-dioxan-2-one typically involves the reaction of tert-butyl alcohol with ethylene carbonate in the presence of a catalyst. The reaction proceeds through a ring-opening mechanism, followed by cyclization to form the dioxane ring. Common catalysts used in this reaction include strong acids such as sulfuric acid or Lewis acids like aluminum chloride.
Industrial Production Methods
In an industrial setting, the production of this compound can be scaled up by optimizing the reaction conditions, such as temperature, pressure, and catalyst concentration. Continuous flow reactors may be employed to enhance the efficiency and yield of the process. The product is typically purified through distillation or recrystallization to achieve the desired purity.
Chemical Reactions Analysis
Types of Reactions
3-Tert-butyl-1,4-dioxan-2-one undergoes various chemical reactions, including:
Oxidation: The compound can be oxidized to form corresponding carbonyl compounds.
Reduction: Reduction reactions can convert the cyclic carbonate into diols or other reduced forms.
Substitution: The tert-butyl group can be substituted with other functional groups through nucleophilic substitution reactions.
Common Reagents and Conditions
Oxidation: Common oxidizing agents include potassium permanganate and chromium trioxide.
Reduction: Reducing agents such as lithium aluminum hydride or sodium borohydride are typically used.
Substitution: Nucleophiles like halides or alkoxides can be used under basic conditions to achieve substitution.
Major Products
The major products formed from these reactions depend on the specific reagents and conditions used. For example, oxidation may yield carbonyl compounds, while reduction can produce diols.
Scientific Research Applications
3-Tert-butyl-1,4-dioxan-2-one has several scientific research applications:
Polymer Chemistry: It is used as a monomer in the synthesis of polycarbonates and polyesters through ring-opening polymerization.
Materials Science: The compound’s unique structure makes it suitable for developing advanced materials with specific properties, such as high thermal stability and mechanical strength.
Biodegradable Polymers: Due to its cyclic carbonate structure, it is explored for creating biodegradable polymers for environmental sustainability.
Drug Delivery Systems: Its biocompatibility and ability to form stable polymers make it a candidate for drug delivery applications.
Mechanism of Action
The mechanism of action of 3-Tert-butyl-1,4-dioxan-2-one in polymerization involves the ring-opening of the cyclic carbonate, followed by the formation of polymer chains. The tert-butyl group provides steric hindrance, which can influence the polymerization kinetics and the properties of the resulting polymer. The molecular targets and pathways involved in its action are primarily related to its reactivity as a cyclic carbonate and its ability to undergo ring-opening reactions.
Comparison with Similar Compounds
Similar Compounds
1,4-Dioxane: A simpler cyclic ether without the tert-butyl group.
Ethylene Carbonate: A cyclic carbonate without the tert-butyl group.
Propylene Carbonate: Another cyclic carbonate with a different alkyl group.
Uniqueness
3-Tert-butyl-1,4-dioxan-2-one is unique due to the presence of the tert-butyl group, which imparts distinct steric and electronic properties. This makes it more resistant to certain reactions and provides specific advantages in polymerization processes, such as improved thermal stability and mechanical properties of the resulting polymers.
Biological Activity
3-Tert-butyl-1,4-dioxan-2-one is a compound that has garnered attention for its potential biological activities, particularly in the fields of antimicrobial and anti-cancer research. This article explores its biological activity, structure-activity relationship (SAR), and relevant case studies, supported by data tables and research findings.
Chemical Structure and Properties
This compound is characterized by a dioxane ring with a tert-butyl substituent, which influences its hydrophobicity and membrane permeability. The molecular formula is C₈H₁₄O₂, and it possesses unique steric properties due to the bulky tert-butyl group. This structural feature is believed to enhance its biological activity by improving its ability to penetrate cellular membranes.
Antimicrobial Activity
Recent studies have highlighted the antimicrobial properties of compounds similar to this compound. For instance, derivatives of tert-butylphenylthiazole , which share structural similarities, have demonstrated significant activity against various bacterial strains, including methicillin-resistant Staphylococcus aureus (MRSA) and Clostridium difficile.
Minimum Inhibitory Concentration (MIC) Values:
Compound | Target Organism | MIC (µg/mL) |
---|---|---|
This compound | MRSA | TBD |
Tert-butylphenylthiazole | MRSA | 4 |
Tert-butylphenylthiazole | C. difficile | 4 |
Tert-butylphenylthiazole | Candida albicans | 4–16 |
The above table summarizes the antimicrobial potency of related compounds. Although specific MIC values for this compound are not yet established, the promising results from similar compounds suggest potential efficacy against resistant strains.
Anti-Cancer Activity
The compound's potential in oncology has also been explored. The bulky tert-butyl group may confer metabolic resistance and enhance cellular uptake, which can be advantageous in cancer therapy. In vitro studies have indicated that derivatives of similar structures exhibit favorable toxicity profiles against cancer cell lines such as MCF-7.
Toxicity Profile:
Compound | Cell Line | Concentration (µg/mL) | Viability (%) |
---|---|---|---|
Tert-butylphenylthiazole | MCF-7 | 32 | 100 |
This data indicates that at high concentrations, certain derivatives maintain cell viability, suggesting a favorable therapeutic index.
Structure-Activity Relationship (SAR)
Understanding the SAR of this compound is crucial for optimizing its biological activity. The presence of the tert-butyl group enhances hydrophobic interactions with lipid membranes, which is critical for antimicrobial efficacy. Studies on related compounds reveal that modifications to the side chains can significantly alter their activity against specific pathogens.
Key Findings:
- Hydrophobicity: Increased hydrophobicity correlates with enhanced membrane penetration.
- Steric Effects: The steric bulk of substituents can hinder metabolic processes, prolonging biological activity.
- Functional Groups: The introduction of polar functional groups can improve solubility without compromising membrane affinity.
Case Studies
A recent study investigated a series of phenylthiazole derivatives with a tert-butyl moiety. These compounds exhibited varying degrees of antimicrobial activity against both Gram-positive and Gram-negative bacteria. Notably, one compound demonstrated an MIC of 4 µg/mL against MRSA and maintained high cell viability in MCF-7 assays at concentrations up to 32 µg/mL.
Properties
IUPAC Name |
3-tert-butyl-1,4-dioxan-2-one |
Source
|
---|---|---|
Details | Computed by LexiChem 2.6.6 (PubChem release 2019.06.18) | |
Source | PubChem | |
URL | https://pubchem.ncbi.nlm.nih.gov | |
Description | Data deposited in or computed by PubChem | |
InChI |
InChI=1S/C8H14O3/c1-8(2,3)6-7(9)11-5-4-10-6/h6H,4-5H2,1-3H3 |
Source
|
Details | Computed by InChI 1.0.5 (PubChem release 2019.06.18) | |
Source | PubChem | |
URL | https://pubchem.ncbi.nlm.nih.gov | |
Description | Data deposited in or computed by PubChem | |
InChI Key |
QWLJHAKWZUZLQP-UHFFFAOYSA-N |
Source
|
Details | Computed by InChI 1.0.5 (PubChem release 2019.06.18) | |
Source | PubChem | |
URL | https://pubchem.ncbi.nlm.nih.gov | |
Description | Data deposited in or computed by PubChem | |
Canonical SMILES |
CC(C)(C)C1C(=O)OCCO1 |
Source
|
Details | Computed by OEChem 2.1.5 (PubChem release 2019.06.18) | |
Source | PubChem | |
URL | https://pubchem.ncbi.nlm.nih.gov | |
Description | Data deposited in or computed by PubChem | |
Molecular Formula |
C8H14O3 |
Source
|
Details | Computed by PubChem 2.1 (PubChem release 2019.06.18) | |
Source | PubChem | |
URL | https://pubchem.ncbi.nlm.nih.gov | |
Description | Data deposited in or computed by PubChem | |
Molecular Weight |
158.19 g/mol |
Source
|
Details | Computed by PubChem 2.1 (PubChem release 2021.05.07) | |
Source | PubChem | |
URL | https://pubchem.ncbi.nlm.nih.gov | |
Description | Data deposited in or computed by PubChem | |
Disclaimer and Information on In-Vitro Research Products
Please be aware that all articles and product information presented on BenchChem are intended solely for informational purposes. The products available for purchase on BenchChem are specifically designed for in-vitro studies, which are conducted outside of living organisms. In-vitro studies, derived from the Latin term "in glass," involve experiments performed in controlled laboratory settings using cells or tissues. It is important to note that these products are not categorized as medicines or drugs, and they have not received approval from the FDA for the prevention, treatment, or cure of any medical condition, ailment, or disease. We must emphasize that any form of bodily introduction of these products into humans or animals is strictly prohibited by law. It is essential to adhere to these guidelines to ensure compliance with legal and ethical standards in research and experimentation.