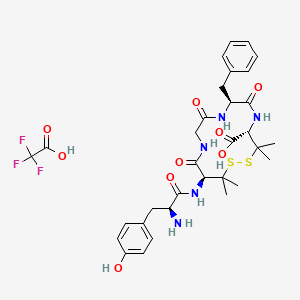
(D-Pen2,D-Pen5)-Enkephalin Trifluoroacetate
- Click on QUICK INQUIRY to receive a quote from our team of experts.
- With the quality product at a COMPETITIVE price, you can focus more on your research.
Description
“(D-Pen2,D-Pen5)-Enkephalin Trifluoroacetate” is a selective agonist of the δ2 opioid receptor . It is a natural stimulant of the opioid receptor, which is widely distributed in the central nervous system and many peripheral tissues of mammals . There are three main types of opioid receptors: μ, δ, and κ .
Molecular Structure Analysis
The molecular structure of “this compound” is complex. Its chemical name is (S)-5-(((®-1-(((S)-1-((2-amino-2-oxoethyl)amino)-3-methyl-1-oxobutan-2-yl)amino)-3-methyl-1-oxobutan-2-yl)amino)-4-(((S)-2-(®-2-(((S)-2-amino-3-(4-hydroxyphenyl)propanamido)propanamido)-3-phenylpropanamido)-5-oxopentanoic acid .Physical And Chemical Properties Analysis
The physical and chemical properties of “this compound” include its appearance as a white lyophilized solid. It has a molecular weight of 782.89 and a CAS number of 122752-16-3. It is soluble to 1 mg/ml in 10% acetonitrile .Mechanism of Action
“(D-Pen2,D-Pen5)-Enkephalin Trifluoroacetate” acts as a selective agonist for the δ opioid receptor . In NG108-15 cells, a short 3-minute treatment with 1 M of this compound significantly reduced the inhibitory effect of the opioid receptor on the generation of cyclic adenosine monophosphate (cAMP), implying that the neuronal δ opioid receptor undergoes rapid desensitization .
Future Directions
Future research could focus on further understanding the roles of the opioid receptor subtypes in opioid agonist-induced effects. This may require comparing the effects of multiple selective agonists in knockout animals . Despite the substantial loss of potency in μ opioid receptor-knockout mice, the synergism of “(D-Pen2,D-Pen5)-Enkephalin Trifluoroacetate” with α2AR agonists is fully retained .
properties
IUPAC Name |
(4S,7S,13S)-13-[[(2S)-2-amino-3-(4-hydroxyphenyl)propanoyl]amino]-7-benzyl-3,3,14,14-tetramethyl-6,9,12-trioxo-1,2-dithia-5,8,11-triazacyclotetradecane-4-carboxylic acid;2,2,2-trifluoroacetic acid |
Source
|
---|---|---|
Details | Computed by Lexichem TK 2.7.0 (PubChem release 2021.05.07) | |
Source | PubChem | |
URL | https://pubchem.ncbi.nlm.nih.gov | |
Description | Data deposited in or computed by PubChem | |
InChI |
InChI=1S/C30H39N5O7S2.C2HF3O2/c1-29(2)23(34-25(38)20(31)14-18-10-12-19(36)13-11-18)27(40)32-16-22(37)33-21(15-17-8-6-5-7-9-17)26(39)35-24(28(41)42)30(3,4)44-43-29;3-2(4,5)1(6)7/h5-13,20-21,23-24,36H,14-16,31H2,1-4H3,(H,32,40)(H,33,37)(H,34,38)(H,35,39)(H,41,42);(H,6,7)/t20-,21-,23-,24-;/m0./s1 |
Source
|
Details | Computed by InChI 1.0.6 (PubChem release 2021.05.07) | |
Source | PubChem | |
URL | https://pubchem.ncbi.nlm.nih.gov | |
Description | Data deposited in or computed by PubChem | |
InChI Key |
LUVILUYVBUMMRG-JZHXPYGKSA-N |
Source
|
Details | Computed by InChI 1.0.6 (PubChem release 2021.05.07) | |
Source | PubChem | |
URL | https://pubchem.ncbi.nlm.nih.gov | |
Description | Data deposited in or computed by PubChem | |
Canonical SMILES |
CC1(C(C(=O)NCC(=O)NC(C(=O)NC(C(SS1)(C)C)C(=O)O)CC2=CC=CC=C2)NC(=O)C(CC3=CC=C(C=C3)O)N)C.C(=O)(C(F)(F)F)O |
Source
|
Details | Computed by OEChem 2.3.0 (PubChem release 2021.05.07) | |
Source | PubChem | |
URL | https://pubchem.ncbi.nlm.nih.gov | |
Description | Data deposited in or computed by PubChem | |
Isomeric SMILES |
CC1([C@H](C(=O)NCC(=O)N[C@H](C(=O)N[C@H](C(SS1)(C)C)C(=O)O)CC2=CC=CC=C2)NC(=O)[C@H](CC3=CC=C(C=C3)O)N)C.C(=O)(C(F)(F)F)O |
Source
|
Details | Computed by OEChem 2.3.0 (PubChem release 2021.05.07) | |
Source | PubChem | |
URL | https://pubchem.ncbi.nlm.nih.gov | |
Description | Data deposited in or computed by PubChem | |
Molecular Formula |
C32H40F3N5O9S2 |
Source
|
Details | Computed by PubChem 2.1 (PubChem release 2021.05.07) | |
Source | PubChem | |
URL | https://pubchem.ncbi.nlm.nih.gov | |
Description | Data deposited in or computed by PubChem | |
Molecular Weight |
759.8 g/mol |
Source
|
Details | Computed by PubChem 2.1 (PubChem release 2021.05.07) | |
Source | PubChem | |
URL | https://pubchem.ncbi.nlm.nih.gov | |
Description | Data deposited in or computed by PubChem | |
Disclaimer and Information on In-Vitro Research Products
Please be aware that all articles and product information presented on BenchChem are intended solely for informational purposes. The products available for purchase on BenchChem are specifically designed for in-vitro studies, which are conducted outside of living organisms. In-vitro studies, derived from the Latin term "in glass," involve experiments performed in controlled laboratory settings using cells or tissues. It is important to note that these products are not categorized as medicines or drugs, and they have not received approval from the FDA for the prevention, treatment, or cure of any medical condition, ailment, or disease. We must emphasize that any form of bodily introduction of these products into humans or animals is strictly prohibited by law. It is essential to adhere to these guidelines to ensure compliance with legal and ethical standards in research and experimentation.