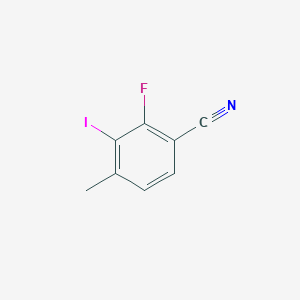
2-Fluoro-3-iodo-4-methylbenzonitrile
Overview
Description
2-Fluoro-3-iodo-4-methylbenzonitrile is an organic compound with the molecular formula C8H5FIN. It is a derivative of benzonitrile, featuring fluorine, iodine, and methyl substituents on the benzene ring. This compound is primarily used in research and development, particularly in the fields of organic synthesis and medicinal chemistry .
Preparation Methods
Synthetic Routes and Reaction Conditions
The synthesis of 2-Fluoro-3-iodo-4-methylbenzonitrile typically involves multi-step organic reactions. One common method is the halogenation of 4-methylbenzonitrile, followed by a substitution reaction to introduce the fluorine and iodine atoms. The reaction conditions often include the use of halogenating agents such as iodine monochloride (ICl) and fluorinating agents like Selectfluor. The reactions are usually carried out in solvents like acetonitrile or dichloromethane under controlled temperatures .
Industrial Production Methods
While specific industrial production methods for this compound are not widely documented, the general approach involves scaling up the laboratory synthesis procedures. This includes optimizing reaction conditions to ensure higher yields and purity, as well as implementing continuous flow processes to enhance efficiency and safety .
Chemical Reactions Analysis
Types of Reactions
2-Fluoro-3-iodo-4-methylbenzonitrile undergoes various chemical reactions, including:
Substitution Reactions: The iodine atom can be replaced by other nucleophiles through nucleophilic substitution reactions.
Coupling Reactions: It can participate in Suzuki-Miyaura and Sonogashira coupling reactions to form carbon-carbon bonds.
Oxidation and Reduction: The compound can be oxidized or reduced under specific conditions to form different derivatives
Common Reagents and Conditions
Nucleophilic Substitution: Reagents like sodium azide or potassium cyanide in polar aprotic solvents.
Coupling Reactions: Palladium catalysts, boronic acids, and bases like potassium carbonate in solvents such as toluene or ethanol.
Oxidation: Oxidizing agents like potassium permanganate or chromium trioxide.
Reduction: Reducing agents like lithium aluminum hydride or sodium borohydride
Major Products Formed
Substitution Products: Various substituted benzonitriles depending on the nucleophile used.
Coupling Products: Biaryl compounds or alkynyl derivatives.
Oxidation and Reduction Products: Different oxidation states of the benzonitrile ring
Scientific Research Applications
Chemical Properties and Structure
- Molecular Formula: CHFIN
- Molecular Weight: 227.05 g/mol
- CAS Number: 147754-12-9
The compound features a nitrile functional group along with halogen substituents, which influence its reactivity and biological activity. The presence of halogens typically increases lipophilicity and metabolic stability, making it a valuable candidate for drug development.
Organic Synthesis
2-Fluoro-3-iodo-4-methylbenzonitrile serves as a crucial building block in the synthesis of more complex organic molecules. It is particularly useful in:
- Substitution Reactions: The iodine atom can be replaced by various nucleophiles, facilitating the formation of diverse substituted benzonitriles.
- Coupling Reactions: It participates in cross-coupling reactions such as Suzuki-Miyaura and Sonogashira reactions to produce biaryl compounds.
Medicinal Chemistry
The compound has been studied for its potential therapeutic applications, including:
- Anticancer Activity: Research indicates that it exhibits cytotoxic effects against various cancer cell lines, potentially through apoptosis induction. For instance, studies have shown significant inhibition of growth in human tumor cells with low micromolar concentrations.
- Antimicrobial Properties: Preliminary evaluations suggest activity against both Gram-positive and Gram-negative bacteria, indicating its potential as a lead compound for new antibiotics .
Chemical Biology
In chemical biology, this compound is utilized as a probe to study biological processes. Its mechanism of action involves:
- Enzyme Inhibition: It has shown promise as an inhibitor of p38 mitogen-activated protein kinase (MAPK), which plays a vital role in inflammatory responses . This inhibition can affect various signaling pathways critical to disease processes.
Case Study 1: Inhibition of p38 MAPK
A study demonstrated that this compound significantly reduced TNF-alpha production in vitro, suggesting potential utility in treating inflammatory diseases .
Case Study 2: Anticancer Properties
Research on various cancer cell lines revealed that this compound exhibited cytotoxic effects at low micromolar concentrations, with mechanisms linked to apoptosis modulation through key signaling pathways .
Case Study 3: Antimicrobial Evaluation
In antimicrobial studies, the compound displayed promising efficacy against multiple pathogens, highlighting its potential for developing new antimicrobial agents .
Mechanism of Action
The mechanism of action of 2-Fluoro-3-iodo-4-methylbenzonitrile depends on its application. In organic synthesis, it acts as a building block, participating in various reactions to form desired products. In medicinal chemistry, its mechanism involves interacting with biological targets, potentially inhibiting or activating specific pathways. The fluorine and iodine atoms can influence the compound’s reactivity and binding affinity to molecular targets .
Comparison with Similar Compounds
Similar Compounds
- 2-Fluoro-4-iodobenzonitrile
- 3-Fluoro-4-methylbenzonitrile
- 2-Fluoro-5-methylbenzonitrile
Uniqueness
2-Fluoro-3-iodo-4-methylbenzonitrile is unique due to the specific positioning of its substituents, which can significantly affect its chemical reactivity and physical properties. The presence of both fluorine and iodine atoms provides a unique combination of electronic effects, making it a valuable intermediate in various synthetic applications .
Biological Activity
2-Fluoro-3-iodo-4-methylbenzonitrile is a halogenated aromatic compound that has garnered attention in medicinal chemistry and biological research due to its potential therapeutic applications. This article explores its biological activity, mechanisms of action, and relevant case studies, providing a comprehensive overview of its significance in scientific research.
This compound has the following chemical structure and properties:
- Molecular Formula: C8H6F I N
- CAS Number: 147754-12-9
- Molecular Weight: 227.05 g/mol
The biological activity of this compound is primarily attributed to its ability to interact with specific molecular targets, such as enzymes and receptors. The presence of fluorine and iodine enhances its binding affinity, potentially influencing various biochemical pathways.
- Enzyme Inhibition : It has been studied for its role as an inhibitor of p38 mitogen-activated protein kinase (MAPK), which is crucial in inflammatory responses and cell signaling pathways .
- Antimicrobial Activity : Preliminary studies suggest that this compound exhibits antimicrobial properties, making it a candidate for further investigation in the development of new antimicrobial agents.
Biological Activity Data
Case Study 1: Inhibition of p38 MAPK
A study evaluated the potential of this compound as a selective inhibitor of p38 MAPK. The compound demonstrated a significant reduction in TNF-alpha production in vitro, suggesting its utility in treating inflammatory diseases .
Case Study 2: Anticancer Properties
Research conducted on various cancer cell lines indicated that this compound exhibited cytotoxic effects, with growth inhibition observed at low micromolar concentrations. The mechanism was linked to its ability to induce apoptosis through modulation of key signaling pathways .
Case Study 3: Antimicrobial Evaluation
In a series of experiments assessing the antimicrobial efficacy of halogenated benzonitriles, this compound showed promising results against both Gram-positive and Gram-negative bacteria. This highlights its potential as a lead compound for developing new antibiotics.
Q & A
Basic Research Questions
Q. What are the optimal synthetic routes for 2-Fluoro-3-iodo-4-methylbenzonitrile, considering iodine’s reactivity?
- Methodology : The iodine substituent’s susceptibility to nucleophilic substitution or elimination necessitates careful selection of reaction conditions. A plausible route involves halogen exchange (e.g., substituting bromine or chlorine with iodine) under controlled temperatures (40–60°C) using NaI in polar aprotic solvents like DMF or DMSO. Precursor molecules like 2-fluoro-3-bromo-4-methylbenzonitrile (analogous to 4-Bromo-2-fluorobenzonitrile in ) can serve as starting materials. Monitor reaction progress via TLC or HPLC to avoid over-iodination or decomposition .
Q. Which spectroscopic techniques are most reliable for characterizing this compound?
- Methodology :
- NMR : NMR identifies fluorine’s electronic environment, while NMR distinguishes nitrile and methyl groups.
- Mass Spectrometry : High-resolution MS (HRMS) confirms molecular weight (e.g., as in for fluorinated analogs).
- X-ray Crystallography : Resolves structural ambiguities, especially steric effects from iodine and methyl groups. Cross-validate data with computational models (e.g., DFT) to address discrepancies in peak assignments .
Q. How should this compound be stored to maintain stability?
- Methodology : Store in amber vials at –20°C under inert gas (argon/nitrogen) to prevent photodegradation of the iodine substituent and hydrolysis of the nitrile group. Conduct accelerated stability studies (40°C/75% RH for 4 weeks) to assess degradation pathways, using HPLC to detect byproducts like benzoic acids or iodides .
Advanced Research Questions
Q. How do fluorine and iodine substituents electronically influence cross-coupling reactivity (e.g., Suzuki-Miyaura)?
- Methodology : Fluorine’s electron-withdrawing effect activates the aryl ring for oxidative addition, while iodine’s polarizability facilitates transmetalation in palladium-catalyzed reactions. Compare reaction rates with analogs lacking iodine (e.g., 3,5-Dichloro-4-fluorobenzonitrile in ) to isolate substituent effects. Use Hammett constants ( for F: +0.34; I: +0.18) to predict regioselectivity in coupling reactions .
Q. What retrosynthetic disconnections are feasible for this compound?
- Methodology :
- Core Disconnection : Split the benzonitrile into iodinated and fluorinated precursors. For example, synthesize 3-iodo-4-methylbenzonitrile first, followed by electrophilic fluorination.
- AI-Driven Synthesis : Tools like Reaxys or Pistachio () can propose routes based on analogous compounds (e.g., 4-Bromo-2-fluorobenzonitrile in ). Validate pathways using small-scale trials and DFT calculations to assess energy barriers .
Q. How can computational models predict regioselectivity in reactions involving this compound?
- Methodology :
- DFT Calculations : Optimize transition states for reactions like nucleophilic aromatic substitution to identify favored positions (e.g., iodine’s para vs. meta sites).
- Molecular Electrostatic Potential (MEP) Maps : Visualize charge distribution to predict attack sites. Validate with kinetic experiments (e.g., competition studies between iodine and fluorine sites) .
Q. Data Contradiction and Validation
Q. How should researchers resolve conflicting spectroscopic data (e.g., unexpected NMR shifts)?
- Methodology :
- Variable Temperature NMR : Assess dynamic effects (e.g., rotational barriers in methyl groups).
- Isotopic Labeling : Substitute with to confirm coupling patterns.
- Comparative Analysis : Cross-reference with structurally similar compounds (e.g., 4-Formyl-3-methoxybenzonitrile in ) to identify anomalies .
Q. What experimental controls are critical when studying this compound’s reactivity?
- Methodology :
- Blank Reactions : Exclude catalysts or reagents to confirm product formation pathways.
- Isotope Trapping : Use / isotopic mixtures to trace iodine’s role in intermediates.
- In Situ Monitoring : Employ techniques like IR spectroscopy to detect transient species (e.g., Pd-I intermediates in cross-coupling) .
Q. Safety and Ethical Considerations
Q. What precautions are essential when handling this compound?
- Methodology : Use fume hoods and personal protective equipment (PPE) due to potential cyanide release from nitrile hydrolysis. Conduct toxicity assays (e.g., Ames test) for waste disposal protocols. Follow guidelines from Safety Data Sheets (SDS) of analogous compounds (e.g., 4-Methoxybenzonitrile in ) .
Properties
IUPAC Name |
2-fluoro-3-iodo-4-methylbenzonitrile | |
---|---|---|
Details | Computed by LexiChem 2.6.6 (PubChem release 2019.06.18) | |
Source | PubChem | |
URL | https://pubchem.ncbi.nlm.nih.gov | |
Description | Data deposited in or computed by PubChem | |
InChI |
InChI=1S/C8H5FIN/c1-5-2-3-6(4-11)7(9)8(5)10/h2-3H,1H3 | |
Details | Computed by InChI 1.0.5 (PubChem release 2019.06.18) | |
Source | PubChem | |
URL | https://pubchem.ncbi.nlm.nih.gov | |
Description | Data deposited in or computed by PubChem | |
InChI Key |
HYKCLWQMKWGJDD-UHFFFAOYSA-N | |
Details | Computed by InChI 1.0.5 (PubChem release 2019.06.18) | |
Source | PubChem | |
URL | https://pubchem.ncbi.nlm.nih.gov | |
Description | Data deposited in or computed by PubChem | |
Canonical SMILES |
CC1=C(C(=C(C=C1)C#N)F)I | |
Details | Computed by OEChem 2.1.5 (PubChem release 2019.06.18) | |
Source | PubChem | |
URL | https://pubchem.ncbi.nlm.nih.gov | |
Description | Data deposited in or computed by PubChem | |
Molecular Formula |
C8H5FIN | |
Details | Computed by PubChem 2.1 (PubChem release 2019.06.18) | |
Source | PubChem | |
URL | https://pubchem.ncbi.nlm.nih.gov | |
Description | Data deposited in or computed by PubChem | |
Molecular Weight |
261.03 g/mol | |
Details | Computed by PubChem 2.1 (PubChem release 2021.05.07) | |
Source | PubChem | |
URL | https://pubchem.ncbi.nlm.nih.gov | |
Description | Data deposited in or computed by PubChem | |
Synthesis routes and methods I
Procedure details
Synthesis routes and methods II
Procedure details
Synthesis routes and methods III
Procedure details
Disclaimer and Information on In-Vitro Research Products
Please be aware that all articles and product information presented on BenchChem are intended solely for informational purposes. The products available for purchase on BenchChem are specifically designed for in-vitro studies, which are conducted outside of living organisms. In-vitro studies, derived from the Latin term "in glass," involve experiments performed in controlled laboratory settings using cells or tissues. It is important to note that these products are not categorized as medicines or drugs, and they have not received approval from the FDA for the prevention, treatment, or cure of any medical condition, ailment, or disease. We must emphasize that any form of bodily introduction of these products into humans or animals is strictly prohibited by law. It is essential to adhere to these guidelines to ensure compliance with legal and ethical standards in research and experimentation.