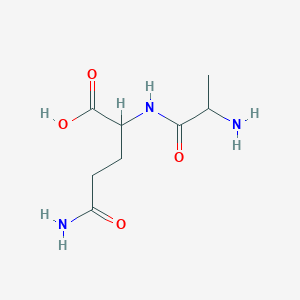
l-Alanyl-l-glutamine
Overview
Description
L-Alanyl-L-glutamine: is a dipeptide composed of the amino acids alanine and glutamine. It is widely used in dietary supplementation, parenteral nutrition, and cell culture due to its high solubility, stability, and bioavailability . This compound is particularly valuable because it provides a stable source of glutamine, which is essential for various physiological functions but is unstable in its free form .
Preparation Methods
Synthetic Routes and Reaction Conditions: L-Alanyl-L-glutamine can be synthesized through chemical and biotechnological methods. One common chemical synthesis method involves the use of L-alanine methyl ester hydrochloride and L-glutamine as substrates in the presence of α-amino acid ester acyltransferase. The reaction is carried out at a pH of 7.0-9.0 and a temperature of 20-40°C .
Industrial Production Methods: Industrial production often employs metabolically engineered strains of Escherichia coli or Pichia pastoris. These strains are modified to overexpress specific enzymes like L-amino acid α-ligase and glutamine synthetase, which facilitate the efficient production of this compound from readily available substrates like glutamic acid and alanine .
Chemical Reactions Analysis
Fermentative Production
Fermentative production using genetically modified Escherichia coli expressing l-amino acid α-ligase (Lal) is another approach. This method involves reducing dipeptide-degrading activity and enhancing substrate amino acid supply. The Lal enzyme catalyzes the formation of dipeptides from unprotected amino acids in an ATP-dependent manner .
Chemical Reactions Involved in Fermentative Production
The fermentative production of this compound involves several key chemical reactions:
-
Dipeptide Synthesis by Lal :
-
Glutamine Biosynthesis :
-
Alanine Dehydrogenase Reaction :
Degradation of this compound
In biological systems, this compound is degraded into its constituent amino acids by peptidases. This process is crucial for providing glutamine to cells, especially in cell culture where free glutamine is unstable .
Scientific Research Applications
Clinical Applications
1. Intensive Care Unit (ICU) Nutrition
L-Alanyl-L-glutamine has been studied for its role in improving outcomes for critically ill patients receiving parenteral nutrition. A randomized trial indicated that supplementation with this compound significantly improved six-month survival rates in ICU patients compared to standard parenteral nutrition. Patients receiving the supplement showed increased plasma glutamine concentrations and reduced mortality rates, suggesting its potential to mitigate glutamine deficiency in severely ill individuals .
2. Lung Preservation During Transplantation
Recent research has explored the use of this compound in ex vivo lung perfusion (EVLP) for donor lung preservation. Modifying the perfusate with this dipeptide demonstrated enhanced cell migration, reduced apoptosis, and improved lung function in porcine models. The study concluded that this compound provides antioxidant and cytoprotective benefits, making it a promising additive for lung preservation protocols .
Metabolic Research
3. Sepsis Management
In animal studies, pre-administration of this compound has been shown to induce significant metabolic changes during sepsis. Rats treated with this dipeptide exhibited increased glycolysis and gluconeogenesis, leading to elevated levels of pyruvate and lactate in the blood and tissues. These findings suggest that this compound may enhance metabolic resilience during septic conditions .
4. Effects on Growth Performance in Aquaculture
This compound supplementation has been investigated for its effects on growth performance in fish species such as Amur sturgeon. A study found that dietary inclusion of this dipeptide improved growth rates and metabolic processes related to nutrient absorption and metabolism, indicating its potential benefits in aquaculture nutrition .
Exercise Physiology
5. Muscle Recovery and Inflammation
The dipeptide has been studied for its effects on muscle recovery post-exercise. Research indicates that supplementation with this compound may reduce muscle damage and inflammation following resistance training. Chronic oral administration was associated with increased levels of heat shock proteins, which play a critical role in cellular protection during stress .
Summary of Key Findings
Mechanism of Action
L-Alanyl-L-glutamine exerts its effects primarily through the release of its constituent amino acids upon hydrolysis. Glutamine, in particular, plays a crucial role in cellular metabolism, protein synthesis, and immune function . It serves as a metabolic fuel for tissues such as the kidney, liver, intestine, heart, and immune cells . The dipeptide form ensures a stable and continuous supply of glutamine, preventing the rapid degradation that occurs with free glutamine .
Comparison with Similar Compounds
Glycyl-L-glutamine: Another dipeptide of glutamine, used similarly in clinical and nutritional applications.
L-Glutamine: The free form of glutamine, which is less stable and more prone to degradation.
Uniqueness: L-Alanyl-L-glutamine is unique due to its high solubility, stability, and bioavailability compared to free L-glutamine. It provides a more reliable source of glutamine in various applications, making it particularly valuable in clinical and research settings .
Q & A
Basic Questions
Q. What experimental considerations are critical when handling L-alanyl-L-glutamine in cell culture studies?
this compound is stable in solution compared to free L-glutamine, which degrades into cytotoxic ammonia. Use it at equimolar concentrations (e.g., 2–4 mM) as a direct substitute. Store lyophilized powder at 2–8°C and reconstituted solutions at -20°C for long-term stability. Sterilize via filtration (0.22 µm) to avoid thermal degradation .
Q. Why is this compound preferred over L-glutamine in cell culture media?
Free L-glutamine undergoes spontaneous hydrolysis in aqueous media, producing ammonia, which inhibits cell growth and viability. The dipeptide form resists degradation, ensuring sustained nutrient availability while reducing ammonia toxicity. For example, Gibco™ CTS™ GlutaMAX™-I directly substitutes L-glutamine at equimolar doses without protocol adjustments .
Q. How does this compound enhance nutrient absorption in cellular models?
The dipeptide is transported via peptide-specific transporters (e.g., PEPT1/2), bypassing competitive uptake mechanisms of free amino acids. This improves intracellular glutamine delivery, supporting redox balance and nucleotide synthesis in rapidly dividing cells like CHO or HEK293 lines .
Advanced Research Questions
Q. What mechanisms underlie this compound’s role in mitigating oxidative stress in immune cells?
In polymorphonuclear leukocytes (PMNs), this compound modulates superoxide anion (O₂⁻) and hydrogen peroxide (H₂O₂) generation by stabilizing glutathione synthesis. A 2002 study showed it reduces myeloperoxidase (MPO) activity by 30% in activated PMNs, suggesting anti-inflammatory potential in sepsis models .
Q. How does this compound preconditioning affect ischemia-reperfusion (I/R) injury outcomes?
In gerbil models of cerebral I/R, preconditioning with 150 mg/kg this compound reduced neuronal apoptosis by 40% via HSP70 upregulation and NF-κB inhibition. Contrastingly, clinical trials in ICU patients reported 20% lower infection rates with dipeptide-supplemented TPN, highlighting translational neuroprotective and metabolic benefits .
Q. Are there discrepancies in clinical vs. preclinical efficacy of this compound?
Preclinical studies emphasize its cytoprotective effects (e.g., 50% reduction in apoptosis post-radiation), while clinical trials focus on metabolic outcomes (e.g., 25% fewer hyperglycemic events in ICU patients). These differences may stem from dosage variations (0.3–0.5 g/kg/day clinically vs. 150–300 mg/kg in rodents) and model-specific endpoints .
Q. How do crystallographic properties of this compound influence pharmaceutical formulations?
New solid forms (Form III, IV, and DMSO solvate) exhibit distinct dissolution profiles. Form III shows 30% faster dissolution than the dihydrate form, enhancing bioavailability in parenteral nutrition. PXRD and Raman spectroscopy are critical for polymorph identification during quality control .
Q. Does this compound synergize with biophysical stimuli in tissue engineering?
Combined with pulsed electrical stimulation (PES), 0.3% this compound increased collagen I/III mRNA expression by 2.5-fold in human dermal fibroblasts. This synergy is attributed to enhanced TGF-β1 secretion and mTOR activation, suggesting utility in chronic wound models .
Q. How does this compound affect cancer cell metabolism in glutamine-deprived conditions?
In glioblastoma cells, the dipeptide restored tricarboxylic acid (TCA) cycle intermediates by 60% under glutamine deprivation, acting as an anaplerotic substrate. However, conflicting data in pancreatic cancer models show Warburg effect potentiation, necessitating context-dependent analysis .
Q. What analytical methods validate this compound purity in pharmaceutical applications?
USP standards recommend HPLC-UV (220 nm, C18 column) with ≥98% purity thresholds. Mass spectrometry confirms molecular integrity (m/z 217.22 for [M+H]⁺), while NMR (¹H/¹³C) identifies stereochemical purity, critical for regulatory compliance in clinical-grade production .
Properties
IUPAC Name |
5-amino-2-(2-aminopropanoylamino)-5-oxopentanoic acid | |
---|---|---|
Details | Computed by LexiChem 2.6.6 (PubChem release 2019.06.18) | |
Source | PubChem | |
URL | https://pubchem.ncbi.nlm.nih.gov | |
Description | Data deposited in or computed by PubChem | |
InChI |
InChI=1S/C8H15N3O4/c1-4(9)7(13)11-5(8(14)15)2-3-6(10)12/h4-5H,2-3,9H2,1H3,(H2,10,12)(H,11,13)(H,14,15) | |
Details | Computed by InChI 1.0.5 (PubChem release 2019.06.18) | |
Source | PubChem | |
URL | https://pubchem.ncbi.nlm.nih.gov | |
Description | Data deposited in or computed by PubChem | |
InChI Key |
HJCMDXDYPOUFDY-UHFFFAOYSA-N | |
Details | Computed by InChI 1.0.5 (PubChem release 2019.06.18) | |
Source | PubChem | |
URL | https://pubchem.ncbi.nlm.nih.gov | |
Description | Data deposited in or computed by PubChem | |
Canonical SMILES |
CC(C(=O)NC(CCC(=O)N)C(=O)O)N | |
Details | Computed by OEChem 2.1.5 (PubChem release 2019.06.18) | |
Source | PubChem | |
URL | https://pubchem.ncbi.nlm.nih.gov | |
Description | Data deposited in or computed by PubChem | |
Molecular Formula |
C8H15N3O4 | |
Details | Computed by PubChem 2.1 (PubChem release 2019.06.18) | |
Source | PubChem | |
URL | https://pubchem.ncbi.nlm.nih.gov | |
Description | Data deposited in or computed by PubChem | |
DSSTOX Substance ID |
DTXSID40865953 | |
Record name | CERAPP_40037 | |
Source | EPA DSSTox | |
URL | https://comptox.epa.gov/dashboard/DTXSID40865953 | |
Description | DSSTox provides a high quality public chemistry resource for supporting improved predictive toxicology. | |
Molecular Weight |
217.22 g/mol | |
Details | Computed by PubChem 2.1 (PubChem release 2021.05.07) | |
Source | PubChem | |
URL | https://pubchem.ncbi.nlm.nih.gov | |
Description | Data deposited in or computed by PubChem | |
Disclaimer and Information on In-Vitro Research Products
Please be aware that all articles and product information presented on BenchChem are intended solely for informational purposes. The products available for purchase on BenchChem are specifically designed for in-vitro studies, which are conducted outside of living organisms. In-vitro studies, derived from the Latin term "in glass," involve experiments performed in controlled laboratory settings using cells or tissues. It is important to note that these products are not categorized as medicines or drugs, and they have not received approval from the FDA for the prevention, treatment, or cure of any medical condition, ailment, or disease. We must emphasize that any form of bodily introduction of these products into humans or animals is strictly prohibited by law. It is essential to adhere to these guidelines to ensure compliance with legal and ethical standards in research and experimentation.