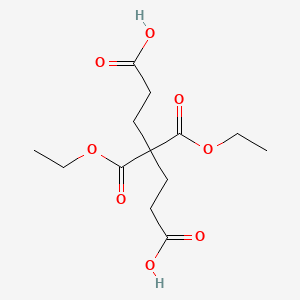
4,4-Bis(ethoxycarbonyl)heptanedioic acid
- Click on QUICK INQUIRY to receive a quote from our team of experts.
- With the quality product at a COMPETITIVE price, you can focus more on your research.
Overview
Description
4,4-Bis(ethoxycarbonyl)heptanedioic acid is an organic compound with the molecular formula C13H20O8 and a molecular weight of 304.3 g/mol . . This compound is characterized by its two ethoxycarbonyl groups attached to a heptanedioic acid backbone, making it a versatile molecule in various chemical applications.
Preparation Methods
The synthesis of 4,4-Bis(ethoxycarbonyl)heptanedioic acid typically involves esterification reactions. One common method includes the reaction of heptanedioic acid with ethanol in the presence of a strong acid catalyst such as sulfuric acid . The reaction conditions usually involve refluxing the mixture to ensure complete esterification. Industrial production methods may involve continuous flow processes to enhance efficiency and yield .
Chemical Reactions Analysis
4,4-Bis(ethoxycarbonyl)heptanedioic acid undergoes various chemical reactions, including:
Oxidation: This compound can be oxidized to form corresponding carboxylic acids.
Reduction: Reduction reactions can convert the ester groups into alcohols.
Substitution: The ethoxycarbonyl groups can be substituted with other functional groups using appropriate reagents.
Common reagents used in these reactions include oxidizing agents like potassium permanganate, reducing agents such as lithium aluminum hydride, and nucleophiles for substitution reactions . Major products formed from these reactions depend on the specific conditions and reagents used.
Scientific Research Applications
4,4-Bis(ethoxycarbonyl)heptanedioic acid has several scientific research applications:
Chemistry: It is used as a building block in organic synthesis for the preparation of more complex molecules.
Biology: The compound can be used in the synthesis of biologically active molecules.
Medicine: It serves as an intermediate in the production of pharmaceuticals.
Industry: The compound is utilized in the manufacture of polymers and other industrial chemicals.
Mechanism of Action
The mechanism of action of 4,4-Bis(ethoxycarbonyl)heptanedioic acid involves its ability to participate in esterification and hydrolysis reactions. The ethoxycarbonyl groups can be hydrolyzed to form carboxylic acids, which can further react with other molecules. The molecular targets and pathways involved depend on the specific application and the chemical environment .
Comparison with Similar Compounds
4,4-Bis(ethoxycarbonyl)heptanedioic acid can be compared with other similar compounds such as:
- 1,2,4-Butanetricarboxylic acid
- 1,3,5-Pentanetricarboxylic acid
- 3,4-Bis(ethoxycarbonyl)-1H-pyrrole-2,5-dicarboxylic acid
- 2,9-Bis-methoxycarbonyl-decanedioic acid dimethyl ester
These compounds share similar structural features but differ in the number and position of carboxyl and ester groups, which influence their reactivity and applications. The uniqueness of this compound lies in its specific arrangement of ethoxycarbonyl groups, making it suitable for particular synthetic and industrial applications .
Biological Activity
4,4-Bis(ethoxycarbonyl)heptanedioic acid, with the chemical formula C13H20O8 and CID 1798255, is a diacid compound featuring two ethoxycarbonyl groups attached to a heptanedioic acid backbone. This compound has garnered attention for its potential biological activities, particularly in medicinal chemistry and organic synthesis. This article explores its biological activity, mechanisms of action, and relevant research findings.
Chemical Structure and Properties
The structure of this compound is characterized by:
- Two ethoxycarbonyl groups : These groups enhance solubility and may influence biological interactions.
- Heptanedioic acid backbone : This provides a flexible chain that can interact with various biological targets.
Property | Value |
---|---|
Molecular Formula | C13H20O8 |
Molecular Weight | 288.30 g/mol |
Melting Point | Not available |
Solubility | Soluble in organic solvents |
The biological activity of this compound is thought to stem from its ability to modulate various signaling pathways within cells. Preliminary studies suggest it may interact with metabolic enzymes, potentially influencing cellular proliferation and apoptosis.
Case Studies and Research Findings
- Anticancer Activity : Recent studies have indicated that derivatives of this compound exhibit significant cytotoxic effects against various cancer cell lines. For instance, a study demonstrated that compounds related to this acid could inhibit the growth of A549 lung cancer cells by targeting metabolic pathways critical for cell survival .
- Metabolic Enzyme Inhibition : Research has highlighted that compounds derived from or related to this compound can inhibit key metabolic enzymes such as NAMPT (Nicotinamide adenine dinucleotide phosphate salvage pathway enzyme), which is crucial for cancer cell metabolism .
- Differential Efficacy in Media Conditions : A comparative analysis revealed that the efficacy of compounds related to this compound varied significantly in different culture media. For example, compounds showed enhanced efficacy in RPMI compared to serum-derived media, indicating the importance of environmental conditions on their biological activity .
Table 2: Summary of Biological Activities
Biological Activity | Observations |
---|---|
Anticancer Effects | Significant cytotoxicity against A549 cells |
Metabolic Pathway Targeting | Inhibition of NAMPT and other metabolic enzymes |
Media Condition Variability | Efficacy varies between RPMI and serum-derived media |
Properties
IUPAC Name |
4,4-bis(ethoxycarbonyl)heptanedioic acid |
Source
|
---|---|---|
Details | Computed by Lexichem TK 2.7.0 (PubChem release 2021.05.07) | |
Source | PubChem | |
URL | https://pubchem.ncbi.nlm.nih.gov | |
Description | Data deposited in or computed by PubChem | |
InChI |
InChI=1S/C13H20O8/c1-3-20-11(18)13(7-5-9(14)15,8-6-10(16)17)12(19)21-4-2/h3-8H2,1-2H3,(H,14,15)(H,16,17) |
Source
|
Details | Computed by InChI 1.0.6 (PubChem release 2021.05.07) | |
Source | PubChem | |
URL | https://pubchem.ncbi.nlm.nih.gov | |
Description | Data deposited in or computed by PubChem | |
InChI Key |
DDMHPCIZAIYLPD-UHFFFAOYSA-N |
Source
|
Details | Computed by InChI 1.0.6 (PubChem release 2021.05.07) | |
Source | PubChem | |
URL | https://pubchem.ncbi.nlm.nih.gov | |
Description | Data deposited in or computed by PubChem | |
Canonical SMILES |
CCOC(=O)C(CCC(=O)O)(CCC(=O)O)C(=O)OCC |
Source
|
Details | Computed by OEChem 2.3.0 (PubChem release 2021.05.07) | |
Source | PubChem | |
URL | https://pubchem.ncbi.nlm.nih.gov | |
Description | Data deposited in or computed by PubChem | |
Molecular Formula |
C13H20O8 |
Source
|
Details | Computed by PubChem 2.1 (PubChem release 2021.05.07) | |
Source | PubChem | |
URL | https://pubchem.ncbi.nlm.nih.gov | |
Description | Data deposited in or computed by PubChem | |
Molecular Weight |
304.29 g/mol |
Source
|
Details | Computed by PubChem 2.1 (PubChem release 2021.05.07) | |
Source | PubChem | |
URL | https://pubchem.ncbi.nlm.nih.gov | |
Description | Data deposited in or computed by PubChem | |
CAS No. |
92454-00-7 |
Source
|
Record name | 4,4-BIS-ETHOXYCARBONYL-HEPTANEDIOIC ACID | |
Source | European Chemicals Agency (ECHA) | |
URL | https://echa.europa.eu/information-on-chemicals | |
Description | The European Chemicals Agency (ECHA) is an agency of the European Union which is the driving force among regulatory authorities in implementing the EU's groundbreaking chemicals legislation for the benefit of human health and the environment as well as for innovation and competitiveness. | |
Explanation | Use of the information, documents and data from the ECHA website is subject to the terms and conditions of this Legal Notice, and subject to other binding limitations provided for under applicable law, the information, documents and data made available on the ECHA website may be reproduced, distributed and/or used, totally or in part, for non-commercial purposes provided that ECHA is acknowledged as the source: "Source: European Chemicals Agency, http://echa.europa.eu/". Such acknowledgement must be included in each copy of the material. ECHA permits and encourages organisations and individuals to create links to the ECHA website under the following cumulative conditions: Links can only be made to webpages that provide a link to the Legal Notice page. | |
Disclaimer and Information on In-Vitro Research Products
Please be aware that all articles and product information presented on BenchChem are intended solely for informational purposes. The products available for purchase on BenchChem are specifically designed for in-vitro studies, which are conducted outside of living organisms. In-vitro studies, derived from the Latin term "in glass," involve experiments performed in controlled laboratory settings using cells or tissues. It is important to note that these products are not categorized as medicines or drugs, and they have not received approval from the FDA for the prevention, treatment, or cure of any medical condition, ailment, or disease. We must emphasize that any form of bodily introduction of these products into humans or animals is strictly prohibited by law. It is essential to adhere to these guidelines to ensure compliance with legal and ethical standards in research and experimentation.