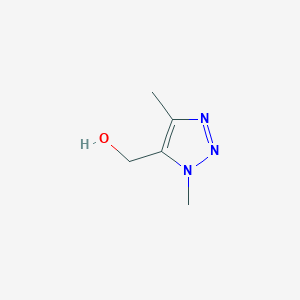
(dimethyl-1H-1,2,3-triazol-5-yl)methanol
Overview
Description
(dimethyl-1H-1,2,3-triazol-5-yl)methanol is an organic compound with the molecular formula C5H9N3O and a molecular weight of 127.15 g/mol . It is a member of the triazole family, which is known for its diverse biological activities and applications in various fields such as chemistry, biology, and medicine .
Preparation Methods
Synthetic Routes and Reaction Conditions
(dimethyl-1H-1,2,3-triazol-5-yl)methanol can be synthesized through various synthetic routes. . This reaction typically occurs under mild conditions and provides high yields of the desired product.
Industrial Production Methods
Industrial production of this compound often involves large-scale synthesis using automated reactors and continuous flow processes. These methods ensure consistent product quality and high efficiency .
Chemical Reactions Analysis
Types of Reactions
(dimethyl-1H-1,2,3-triazol-5-yl)methanol undergoes various chemical reactions, including:
Oxidation: The hydroxyl group can be oxidized to form the corresponding aldehyde or carboxylic acid.
Reduction: The triazole ring can be reduced under specific conditions to form different derivatives.
Substitution: The hydroxyl group can be substituted with other functional groups, such as halides or amines.
Common Reagents and Conditions
Common reagents used in these reactions include oxidizing agents like potassium permanganate, reducing agents like lithium aluminum hydride, and halogenating agents like thionyl chloride .
Major Products Formed
The major products formed from these reactions include various triazole derivatives, which can be further functionalized for specific applications .
Scientific Research Applications
Medicinal Chemistry
Antimicrobial Properties
Research indicates that triazole derivatives, including (dimethyl-1H-1,2,3-triazol-5-yl)methanol, exhibit significant antimicrobial activity. A study highlighted the compound's effectiveness against various bacterial strains, demonstrating its potential as an antibacterial agent. The presence of the triazole ring enhances its interaction with biological targets, making it a candidate for further development in pharmaceuticals aimed at treating infections caused by resistant bacteria .
Antifungal Activity
Triazole compounds are well-known for their antifungal properties. This compound can inhibit the growth of fungi by interfering with ergosterol synthesis, a vital component of fungal cell membranes. This mechanism is crucial for developing new antifungal medications to combat fungal infections that are increasingly resistant to existing treatments .
Agriculture
Plant Growth Regulators
The application of this compound as a plant growth regulator has been investigated. Its ability to modulate plant growth and development could lead to enhanced agricultural productivity. Studies have shown that triazole compounds can improve stress tolerance in plants by regulating hormonal pathways and enhancing nutrient uptake .
Pesticide Development
The compound's structural features make it a suitable candidate for designing novel pesticides. Research has demonstrated that triazole derivatives can exhibit insecticidal and fungicidal activities. The incorporation of this compound into pesticide formulations could enhance efficacy against pests while minimizing environmental impact .
Material Science
Synthesis of Coordination Polymers
this compound has been used in the synthesis of coordination polymers and metal-organic frameworks (MOFs). These materials have applications in gas storage and separation due to their high surface area and tunable porosity. The incorporation of triazole ligands enhances the stability and functionality of these frameworks .
Catalysis
The compound has shown potential as a catalyst in various organic reactions. Its ability to stabilize transition states makes it effective in promoting chemical transformations. Research has indicated that triazole-based catalysts can facilitate reactions such as cross-coupling and oxidation processes with high efficiency .
Case Studies
Mechanism of Action
The mechanism of action of (dimethyl-1H-1,2,3-triazol-5-yl)methanol involves its interaction with specific molecular targets and pathways. For example, it can inhibit certain enzymes or bind to specific receptors, leading to various biological effects . The triazole ring is known to interact with metal ions and other biomolecules, which can modulate its activity .
Comparison with Similar Compounds
(dimethyl-1H-1,2,3-triazol-5-yl)methanol can be compared with other similar compounds, such as:
(1,3-dimethyl-1H-1,2,4-triazol-5-yl)methanol: Similar structure but different substitution pattern on the triazole ring.
(1-benzyl-1H-1,2,3-triazol-5-yl)methanol: Contains a benzyl group instead of dimethyl groups, leading to different chemical and biological properties.
These comparisons highlight the uniqueness of this compound in terms of its specific substitution pattern and resulting properties.
Biological Activity
(dimethyl-1H-1,2,3-triazol-5-yl)methanol is an organic compound with the molecular formula CHNO and a molecular weight of 127.15 g/mol. It has garnered attention in scientific research for its potential biological activities, particularly in the fields of antimicrobial and antiviral research, as well as its applications in drug development.
The compound can be synthesized through various methods, including reactions involving azides and alkynes via "Click" chemistry. It can also undergo several chemical transformations such as oxidation and substitution, which may enhance its biological activity by modifying its functional groups .
The biological activity of this compound is primarily attributed to its ability to interact with specific molecular targets. This compound may inhibit certain enzymes or bind to receptors involved in various biochemical pathways. For example, its structural similarity to other triazole compounds suggests potential interactions with fungal cytochrome P450 enzymes, which are critical for fungal growth and survival .
Antimicrobial Properties
Research indicates that this compound exhibits significant antimicrobial activity against a range of pathogens. Studies have demonstrated its effectiveness against both Gram-positive and Gram-negative bacteria, as well as fungi. The compound's mechanism likely involves disrupting microbial cell wall synthesis or interfering with metabolic pathways essential for microbial survival .
Antiviral Activity
In addition to its antibacterial properties, this compound has shown promise as an antiviral agent. Preliminary studies suggest that it may inhibit viral replication by targeting viral enzymes or interfering with the host cell's machinery necessary for viral propagation .
Anticancer Potential
The compound has also been explored for its anticancer properties. In vitro studies have indicated that this compound can induce apoptosis in cancer cell lines. Its mechanism may involve the activation of caspases and modulation of signaling pathways related to cell survival and proliferation .
Case Studies
Case Study 1: Antimicrobial Efficacy
A study evaluated the antimicrobial efficacy of this compound against various bacterial strains. The minimum inhibitory concentration (MIC) values were determined using standard broth microdilution methods. Results indicated that the compound exhibited MIC values ranging from 8 to 32 µg/mL against tested strains.
Bacterial Strain | MIC (µg/mL) |
---|---|
Staphylococcus aureus | 16 |
Escherichia coli | 32 |
Candida albicans | 8 |
Case Study 2: Antiviral Activity
In a separate investigation focusing on antiviral activity, this compound was tested against influenza virus. The compound demonstrated a dose-dependent reduction in viral titers in infected cell cultures.
Concentration (µM) | Viral Titer Reduction (%) |
---|---|
10 | 25 |
25 | 50 |
50 | 75 |
Q & A
Basic Research Questions
Q. What are the common synthetic routes for (dimethyl-1H-1,2,3-triazol-5-yl)methanol?
The compound is typically synthesized via copper(I)-catalyzed azide-alkyne cycloaddition (CuAAC), a regioselective method for 1,2,3-triazole formation. For example, terminal alkynes react with azides under mild conditions to yield 1,4-disubstituted triazoles . Post-synthetic modifications, such as methylation or hydroxylation, can introduce the dimethyl and methanol groups. Solvent choice (e.g., DMF or aqueous mixtures) and catalyst loading (e.g., CuSO₄·5H₂O with sodium ascorbate) are critical for yield optimization.
Q. How is the compound characterized using spectroscopic and crystallographic methods?
- Spectroscopy : ¹H and ¹³C NMR confirm substituent positions and regiochemistry. The hydroxyl proton in the methanol group appears as a broad singlet (~1–5 ppm), while triazole protons resonate between 7.5–8.5 ppm.
- Crystallography : Single-crystal X-ray diffraction (SC-XRD) with programs like SHELXL ( ) resolves the 3D structure. For example, PDB entries 5s9r and 7mce ( ) illustrate triazole-containing ligands in complex with BRD4, highlighting bond angles and packing interactions .
Q. What are the typical applications of this compound in supramolecular or coordination chemistry?
The triazole ring acts as a versatile ligand for metal coordination (e.g., Cu²⁺, Zn²⁺) in metallogels or frameworks. demonstrates that triazole-carboxylic acid derivatives form stable coordination polymers, suggesting analogous applications for this compound in designing functional materials .
Advanced Research Questions
Q. How can crystallographic disorder in the triazole ring be addressed during refinement?
Disorder arises from dynamic motion or overlapping electron density. SHELXL’s PART and EADP commands ( ) refine partial occupancies, while DFIX and SADI restraints maintain geometric consistency. For example, rotational disorder in the dimethyl group can be modeled using split positions with occupancy constraints .
Q. What computational strategies are employed to study electronic properties and regiochemical outcomes?
Density Functional Theory (DFT) at the B3LYP/6-311++G(d,p) level calculates optimized geometries, frontier molecular orbitals (HOMO-LUMO), and electrostatic potentials. and highlight DFT’s role in correlating triazole substitution patterns (e.g., 1,2,3- vs. 1,2,4-triazole) with stability and reactivity .
Q. How does regioselectivity in triazole synthesis impact biological activity?
The 1,2,3-triazol-5-yl isomer (vs. 1,2,3-triazol-4-yl) influences binding interactions in enzymatic pockets. For instance, shows that triazole connectivity affects inhibitory potency in acetylcholinesterase complexes. Molecular docking (e.g., AutoDock Vina) can predict binding modes of the methanol-substituted derivative .
Q. What experimental and theoretical methods resolve contradictions in spectroscopic vs. crystallographic data?
Discrepancies between NMR (solution state) and SC-XRD (solid state) arise from conformational flexibility. Hybrid approaches include:
- Variable-temperature NMR to probe dynamic behavior.
- Periodic DFT calculations (e.g., CASTEP) to compare solid-state and gas-phase geometries .
Q. Methodological Notes
- Synthesis Optimization : Use high-throughput screening (e.g., parallel reactions in microplates) to test azide/alkyne ratios and catalyst systems.
- Crystallization : Co-crystallize with bromodomains (as in ) for structural biology applications.
- Data Validation : Cross-validate computational results with experimental SC-XRD and spectroscopic data to ensure accuracy.
Properties
IUPAC Name |
(3,5-dimethyltriazol-4-yl)methanol | |
---|---|---|
Details | Computed by LexiChem 2.6.6 (PubChem release 2019.06.18) | |
Source | PubChem | |
URL | https://pubchem.ncbi.nlm.nih.gov | |
Description | Data deposited in or computed by PubChem | |
InChI |
InChI=1S/C5H9N3O/c1-4-5(3-9)8(2)7-6-4/h9H,3H2,1-2H3 | |
Details | Computed by InChI 1.0.5 (PubChem release 2019.06.18) | |
Source | PubChem | |
URL | https://pubchem.ncbi.nlm.nih.gov | |
Description | Data deposited in or computed by PubChem | |
InChI Key |
QVDPIHKJPWCHTP-UHFFFAOYSA-N | |
Details | Computed by InChI 1.0.5 (PubChem release 2019.06.18) | |
Source | PubChem | |
URL | https://pubchem.ncbi.nlm.nih.gov | |
Description | Data deposited in or computed by PubChem | |
Canonical SMILES |
CC1=C(N(N=N1)C)CO | |
Details | Computed by OEChem 2.1.5 (PubChem release 2019.06.18) | |
Source | PubChem | |
URL | https://pubchem.ncbi.nlm.nih.gov | |
Description | Data deposited in or computed by PubChem | |
Molecular Formula |
C5H9N3O | |
Details | Computed by PubChem 2.1 (PubChem release 2019.06.18) | |
Source | PubChem | |
URL | https://pubchem.ncbi.nlm.nih.gov | |
Description | Data deposited in or computed by PubChem | |
Molecular Weight |
127.14 g/mol | |
Details | Computed by PubChem 2.1 (PubChem release 2021.05.07) | |
Source | PubChem | |
URL | https://pubchem.ncbi.nlm.nih.gov | |
Description | Data deposited in or computed by PubChem | |
Disclaimer and Information on In-Vitro Research Products
Please be aware that all articles and product information presented on BenchChem are intended solely for informational purposes. The products available for purchase on BenchChem are specifically designed for in-vitro studies, which are conducted outside of living organisms. In-vitro studies, derived from the Latin term "in glass," involve experiments performed in controlled laboratory settings using cells or tissues. It is important to note that these products are not categorized as medicines or drugs, and they have not received approval from the FDA for the prevention, treatment, or cure of any medical condition, ailment, or disease. We must emphasize that any form of bodily introduction of these products into humans or animals is strictly prohibited by law. It is essential to adhere to these guidelines to ensure compliance with legal and ethical standards in research and experimentation.