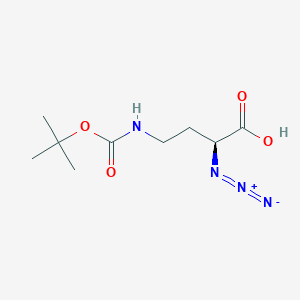
N3-L-Dab(Boc)-OH
- Click on QUICK INQUIRY to receive a quote from our team of experts.
- With the quality product at a COMPETITIVE price, you can focus more on your research.
Overview
Description
N3-L-Dab(Boc)-OH: is a chemical compound known as N3-L-2,4-diaminobutyric acid (Boc)-OH. It is a derivative of the amino acid 2,4-diaminobutyric acid, which is modified with a tert-butoxycarbonyl (Boc) protecting group. This compound is commonly used in peptide synthesis and other chemical research applications due to its unique properties.
Preparation Methods
Synthetic Routes and Reaction Conditions:
Starting Materials: The synthesis of N3-L-2,4-diaminobutyric acid (Boc)-OH typically begins with the amino acid 2,4-diaminobutyric acid.
Protection of Amino Groups: The amino groups of 2,4-diaminobutyric acid are protected using a tert-butoxycarbonyl (Boc) group. This is achieved by reacting the amino acid with di-tert-butyl dicarbonate (Boc2O) in the presence of a base such as triethylamine.
Purification: The resulting product is purified using techniques such as recrystallization or chromatography to obtain pure N3-L-2,4-diaminobutyric acid (Boc)-OH.
Industrial Production Methods: Industrial production of N3-L-2,4-diaminobutyric acid (Boc)-OH follows similar synthetic routes but on a larger scale. The process involves the use of automated peptide synthesizers and large-scale purification methods to ensure high yield and purity.
Chemical Reactions Analysis
Types of Reactions:
Oxidation: N3-L-2,4-diaminobutyric acid (Boc)-OH can undergo oxidation reactions to form various oxidized derivatives.
Reduction: The compound can be reduced to form different reduced derivatives.
Substitution: It can participate in substitution reactions where the Boc protecting group is replaced with other functional groups.
Common Reagents and Conditions:
Oxidation Reagents: Common oxidation reagents include hydrogen peroxide and potassium permanganate.
Reduction Reagents: Reduction can be achieved using reagents such as sodium borohydride or lithium aluminum hydride.
Substitution Reagents: Substitution reactions often involve reagents like trifluoroacetic acid (TFA) to remove the Boc protecting group.
Major Products Formed: The major products formed from these reactions depend on the specific reagents and conditions used. For example, oxidation may yield oxidized derivatives, while reduction may produce reduced derivatives.
Scientific Research Applications
Chemistry: N3-L-2,4-diaminobutyric acid (Boc)-OH is widely used in peptide synthesis as a building block for creating peptides and proteins. It is also used in the development of new chemical reactions and methodologies.
Biology: In biological research, this compound is used to study the structure and function of proteins and peptides. It is also employed in the synthesis of peptide-based drugs and biomolecules.
Medicine: N3-L-2,4-diaminobutyric acid (Boc)-OH is used in the development of peptide-based therapeutics. It is also utilized in drug discovery and development processes.
Industry: In the industrial sector, this compound is used in the production of various chemicals and materials. It is also employed in the manufacturing of pharmaceuticals and biotechnology products.
Mechanism of Action
The mechanism of action of N3-L-2,4-diaminobutyric acid (Boc)-OH involves its role as a building block in peptide synthesis. The Boc protecting group helps to protect the amino groups during chemical reactions, allowing for selective modification of the molecule. The compound interacts with various molecular targets and pathways depending on its specific application.
Comparison with Similar Compounds
N3-L-2,4-diaminobutyric acid: The unprotected form of the compound.
N3-L-2,4-diaminobutyric acid (Fmoc)-OH: A similar compound with a different protecting group (fluorenylmethyloxycarbonyl).
N3-L-2,4-diaminobutyric acid (Cbz)-OH: Another similar compound with a carbobenzyloxy protecting group.
Uniqueness: N3-L-2,4-diaminobutyric acid (Boc)-OH is unique due to its specific Boc protecting group, which provides stability and selectivity during chemical reactions. This makes it particularly useful in peptide synthesis and other chemical research applications.
Biological Activity
N3-L-Dab(Boc)-OH, a derivative of diaminobutyric acid, has garnered attention in the fields of peptide synthesis, drug development, and bioconjugation due to its unique structural properties and biological activities. This article explores the compound's biological activity, applications, and relevant research findings.
- Chemical Name : this compound
- Molecular Formula : C24H28N2O4
- Molecular Weight : 440.5 g/mol
- CAS Number : 125238-99-5
- Purity : Minimum 99% .
Applications in Research
This compound is primarily utilized in several key areas:
-
Peptide Synthesis :
- Functions as a building block for modified peptides that enhance therapeutic efficacy.
- Facilitates the incorporation of azide functionalities for click chemistry applications.
-
Drug Development :
- Plays a crucial role in designing prodrugs aimed at improving bioavailability and minimizing side effects.
- Used in the synthesis of peptide-based therapeutics targeting various diseases.
-
Bioconjugation :
- Essential in attaching biomolecules to surfaces or other molecules, enabling targeted drug delivery systems.
- Enhances the stability and efficacy of drug formulations through conjugation strategies.
- Chemical Biology :
Case Studies
-
Peptide Therapeutics Development :
A study demonstrated that peptides synthesized using this compound exhibited enhanced binding affinity to target receptors compared to traditional amino acids. This was particularly noted in the development of peptides targeting cancer cells, where modifications led to improved cellular uptake and therapeutic outcomes . -
Bioconjugation Techniques :
Research highlighted the use of this compound in bioconjugation with monoclonal antibodies, resulting in stable immunoconjugates with significant anti-tumor activity in preclinical models. The conjugates showed a marked increase in specificity towards tumor cells while minimizing off-target effects . -
Toxicity and Efficacy Studies :
In vitro studies assessed the cytotoxic effects of drug candidates synthesized with this compound on various cancer cell lines. Results indicated that these compounds had a dose-dependent cytotoxic effect, effectively reducing cell viability while maintaining acceptable safety profiles .
Data Table: Summary of Biological Activities
Properties
IUPAC Name |
(2S)-2-azido-4-[(2-methylpropan-2-yl)oxycarbonylamino]butanoic acid |
Source
|
---|---|---|
Details | Computed by LexiChem 2.6.6 (PubChem release 2019.06.18) | |
Source | PubChem | |
URL | https://pubchem.ncbi.nlm.nih.gov | |
Description | Data deposited in or computed by PubChem | |
InChI |
InChI=1S/C9H16N4O4/c1-9(2,3)17-8(16)11-5-4-6(7(14)15)12-13-10/h6H,4-5H2,1-3H3,(H,11,16)(H,14,15)/t6-/m0/s1 |
Source
|
Details | Computed by InChI 1.0.5 (PubChem release 2019.06.18) | |
Source | PubChem | |
URL | https://pubchem.ncbi.nlm.nih.gov | |
Description | Data deposited in or computed by PubChem | |
InChI Key |
JLMWKZYQNHSSAD-LURJTMIESA-N |
Source
|
Details | Computed by InChI 1.0.5 (PubChem release 2019.06.18) | |
Source | PubChem | |
URL | https://pubchem.ncbi.nlm.nih.gov | |
Description | Data deposited in or computed by PubChem | |
Canonical SMILES |
CC(C)(C)OC(=O)NCCC(C(=O)O)N=[N+]=[N-] |
Source
|
Details | Computed by OEChem 2.1.5 (PubChem release 2019.06.18) | |
Source | PubChem | |
URL | https://pubchem.ncbi.nlm.nih.gov | |
Description | Data deposited in or computed by PubChem | |
Isomeric SMILES |
CC(C)(C)OC(=O)NCC[C@@H](C(=O)O)N=[N+]=[N-] |
Source
|
Details | Computed by OEChem 2.1.5 (PubChem release 2019.06.18) | |
Source | PubChem | |
URL | https://pubchem.ncbi.nlm.nih.gov | |
Description | Data deposited in or computed by PubChem | |
Molecular Formula |
C9H16N4O4 |
Source
|
Details | Computed by PubChem 2.1 (PubChem release 2019.06.18) | |
Source | PubChem | |
URL | https://pubchem.ncbi.nlm.nih.gov | |
Description | Data deposited in or computed by PubChem | |
Molecular Weight |
244.25 g/mol |
Source
|
Details | Computed by PubChem 2.1 (PubChem release 2021.05.07) | |
Source | PubChem | |
URL | https://pubchem.ncbi.nlm.nih.gov | |
Description | Data deposited in or computed by PubChem | |
Disclaimer and Information on In-Vitro Research Products
Please be aware that all articles and product information presented on BenchChem are intended solely for informational purposes. The products available for purchase on BenchChem are specifically designed for in-vitro studies, which are conducted outside of living organisms. In-vitro studies, derived from the Latin term "in glass," involve experiments performed in controlled laboratory settings using cells or tissues. It is important to note that these products are not categorized as medicines or drugs, and they have not received approval from the FDA for the prevention, treatment, or cure of any medical condition, ailment, or disease. We must emphasize that any form of bodily introduction of these products into humans or animals is strictly prohibited by law. It is essential to adhere to these guidelines to ensure compliance with legal and ethical standards in research and experimentation.