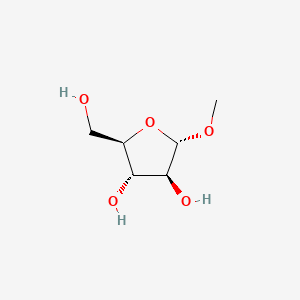
Methyl Alpha-D-Arabinofuranoside
- Click on QUICK INQUIRY to receive a quote from our team of experts.
- With the quality product at a COMPETITIVE price, you can focus more on your research.
Overview
Description
Methyl Alpha-D-Arabinofuranoside is a chemical compound with the molecular formula C6H12O5 It is a derivative of arabinose, a five-carbon sugar, and exists in the furanose form
Preparation Methods
Synthetic Routes and Reaction Conditions: Methyl Alpha-D-Arabinofuranoside can be synthesized through the methylation of arabinose. The process typically involves the use of methanol and an acid catalyst to facilitate the reaction. The reaction conditions often include heating the mixture to promote the formation of the furanoside ring structure.
Industrial Production Methods: Industrial production of this compound may involve similar synthetic routes but on a larger scale. The use of continuous flow reactors and optimized reaction conditions can enhance yield and efficiency. Purification steps such as crystallization or chromatography are employed to obtain the desired purity.
Chemical Reactions Analysis
Types of Reactions: Methyl Alpha-D-Arabinofuranoside undergoes various chemical reactions, including:
Oxidation: The hydroxyl groups can be oxidized to form corresponding aldehydes or carboxylic acids.
Reduction: Reduction reactions can convert the compound into its corresponding alcohols.
Substitution: The hydroxyl groups can be substituted with other functional groups using appropriate reagents.
Common Reagents and Conditions:
Oxidation: Common oxidizing agents include potassium permanganate (KMnO4) and chromium trioxide (CrO3).
Reduction: Reducing agents such as sodium borohydride (NaBH4) or lithium aluminum hydride (LiAlH4) are used.
Substitution: Reagents like acyl chlorides or alkyl halides can be used for substitution reactions.
Major Products Formed:
Oxidation: Formation of arabinonic acid or arabinose derivatives.
Reduction: Formation of arabinitol.
Substitution: Formation of various substituted arabinofuranosides.
Scientific Research Applications
Methyl Alpha-D-Arabinofuranoside has diverse applications in scientific research:
Chemistry: Used as a building block in the synthesis of complex carbohydrates and glycosides.
Biology: Serves as a substrate for studying enzyme activities, particularly those involved in carbohydrate metabolism.
Industry: Utilized in the production of biofuels and as a precursor for various fine chemicals.
Mechanism of Action
The mechanism of action of Methyl Alpha-D-Arabinofuranoside involves its interaction with specific enzymes and molecular targets. In biological systems, it can be hydrolyzed by enzymes such as arabinofuranosidases, leading to the release of arabinose. This process is crucial in the metabolism of arabinose-containing polysaccharides. The compound’s effects are mediated through its participation in metabolic pathways involving carbohydrate processing.
Comparison with Similar Compounds
- Methyl Beta-D-Arabinofuranoside
- Methyl Alpha-D-Xylopyranoside
- Methyl Beta-D-Xylopyranoside
Comparison: Methyl Alpha-D-Arabinofuranoside is unique due to its specific furanose ring structure and the configuration of its hydroxyl groups. Compared to its beta-anomer, it exhibits different reactivity and interaction with enzymes. The presence of the furanose ring distinguishes it from pyranose forms like Methyl Alpha-D-Xylopyranoside, which have a six-membered ring structure.
Properties
IUPAC Name |
(2R,3S,4S,5S)-2-(hydroxymethyl)-5-methoxyoxolane-3,4-diol |
Source
|
---|---|---|
Details | Computed by Lexichem TK 2.7.0 (PubChem release 2021.05.07) | |
Source | PubChem | |
URL | https://pubchem.ncbi.nlm.nih.gov | |
Description | Data deposited in or computed by PubChem | |
InChI |
InChI=1S/C6H12O5/c1-10-6-5(9)4(8)3(2-7)11-6/h3-9H,2H2,1H3/t3-,4-,5+,6+/m1/s1 |
Source
|
Details | Computed by InChI 1.0.6 (PubChem release 2021.05.07) | |
Source | PubChem | |
URL | https://pubchem.ncbi.nlm.nih.gov | |
Description | Data deposited in or computed by PubChem | |
InChI Key |
NALRCAPFICWVAQ-ZXXMMSQZSA-N |
Source
|
Details | Computed by InChI 1.0.6 (PubChem release 2021.05.07) | |
Source | PubChem | |
URL | https://pubchem.ncbi.nlm.nih.gov | |
Description | Data deposited in or computed by PubChem | |
Canonical SMILES |
COC1C(C(C(O1)CO)O)O |
Source
|
Details | Computed by OEChem 2.3.0 (PubChem release 2021.05.07) | |
Source | PubChem | |
URL | https://pubchem.ncbi.nlm.nih.gov | |
Description | Data deposited in or computed by PubChem | |
Isomeric SMILES |
CO[C@@H]1[C@H]([C@@H]([C@H](O1)CO)O)O |
Source
|
Details | Computed by OEChem 2.3.0 (PubChem release 2021.05.07) | |
Source | PubChem | |
URL | https://pubchem.ncbi.nlm.nih.gov | |
Description | Data deposited in or computed by PubChem | |
Molecular Formula |
C6H12O5 |
Source
|
Details | Computed by PubChem 2.1 (PubChem release 2021.05.07) | |
Source | PubChem | |
URL | https://pubchem.ncbi.nlm.nih.gov | |
Description | Data deposited in or computed by PubChem | |
Molecular Weight |
164.16 g/mol |
Source
|
Details | Computed by PubChem 2.1 (PubChem release 2021.05.07) | |
Source | PubChem | |
URL | https://pubchem.ncbi.nlm.nih.gov | |
Description | Data deposited in or computed by PubChem | |
Disclaimer and Information on In-Vitro Research Products
Please be aware that all articles and product information presented on BenchChem are intended solely for informational purposes. The products available for purchase on BenchChem are specifically designed for in-vitro studies, which are conducted outside of living organisms. In-vitro studies, derived from the Latin term "in glass," involve experiments performed in controlled laboratory settings using cells or tissues. It is important to note that these products are not categorized as medicines or drugs, and they have not received approval from the FDA for the prevention, treatment, or cure of any medical condition, ailment, or disease. We must emphasize that any form of bodily introduction of these products into humans or animals is strictly prohibited by law. It is essential to adhere to these guidelines to ensure compliance with legal and ethical standards in research and experimentation.