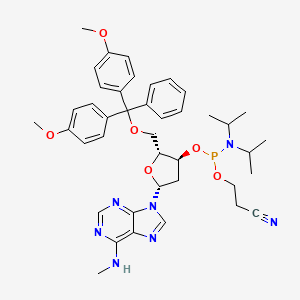
N6-Methyl-dAphosphoramidite
Overview
Description
N6-Methyl-dA phosphoramidite is a modified nucleoside used in the synthesis of oligonucleotides. It is a derivative of deoxyadenosine, where a methyl group is attached to the nitrogen at the sixth position of the adenine base. This modification is significant in the study of DNA methylation and epigenetics, as it mimics the natural methylation process that occurs in biological systems .
Mechanism of Action
Target of Action
N6-Methyl-dAphosphoramidite is primarily used in the synthesis of oligodeoxyribonucleotides . Oligodeoxyribonucleotides are short DNA molecules that play crucial roles in research, diagnostics, and therapeutics .
Mode of Action
This compound interacts with its targets, the oligodeoxyribonucleotides, through a process known as methylation . Methylation is a biological process where a methyl group is added to a molecule, in this case, the adenine of the oligodeoxyribonucleotide . This methylation can affect the function and behavior of the oligodeoxyribonucleotides .
Biochemical Pathways
The biochemical pathways affected by this compound are those involved in the synthesis and function of oligodeoxyribonucleotides . The methylation of adenine can influence various RNA metabolism processes that affect the fate of cells, including cancer development . Therefore, the pathways affected by this compound could have significant downstream effects on cellular function and disease progression .
Pharmacokinetics
It’s known that the compound is used in vitro and is soluble in dmso . Its bioavailability would be dependent on the method of delivery when used in research or therapeutic applications .
Result of Action
The molecular and cellular effects of this compound’s action are primarily seen in its role in the synthesis of methylated oligodeoxyribonucleotides . The methylation of adenine in these molecules can influence their stability, splicing, export, translation, and decay . These changes can have significant effects on cellular function and could potentially be leveraged for therapeutic purposes .
Action Environment
The action, efficacy, and stability of this compound can be influenced by various environmental factors. For instance, the compound should be stored at 4°C, away from moisture and light, to maintain its stability . Furthermore, the compound’s solubility can be affected by the solvent used, with DMSO being recommended . These factors must be carefully controlled to ensure the effective use of this compound in the synthesis of oligodeoxyribonucleotides .
Biochemical Analysis
Biochemical Properties
N6-Methyl-dAphosphoramidite interacts with various enzymes, proteins, and other biomolecules in the process of oligodeoxyribonucleotide synthesis . The nature of these interactions is primarily chemical, involving the exchange and sharing of electrons during the formation of covalent bonds. The compound’s role in these biochemical reactions is crucial as it contributes to the formation of the phosphoramidite moiety, which is highly reactive towards nucleophiles .
Cellular Effects
The effects of this compound on cells and cellular processes are primarily related to its role in the synthesis of oligodeoxyribonucleotides These oligodeoxyribonucleotides can influence cell function by impacting cell signaling pathways, gene expression, and cellular metabolism
Molecular Mechanism
The molecular mechanism of action of this compound involves its participation in the synthesis of oligodeoxyribonucleotides . It exerts its effects at the molecular level through binding interactions with biomolecules, contributing to enzyme activation during the synthesis process . Changes in gene expression may also occur as a result of the incorporation of the synthesized oligodeoxyribonucleotides into the cellular machinery.
Temporal Effects in Laboratory Settings
The temporal effects of this compound in laboratory settings are largely related to its stability and degradation over time. As a chemical compound used in the synthesis of oligodeoxyribonucleotides, it is expected to remain stable under appropriate storage conditions
Metabolic Pathways
This compound is involved in the metabolic pathway of oligodeoxyribonucleotide synthesis This process involves a series of enzymatic reactions, with this compound serving as a key intermediate The compound may interact with various enzymes or cofactors during this process
Transport and Distribution
The transport and distribution of this compound within cells and tissues are likely to be influenced by its chemical properties and the specific cellular mechanisms involved in oligodeoxyribonucleotide synthesis . Detailed information on any transporters or binding proteins that it interacts with, as well as any effects on its localization or accumulation, is currently lacking.
Subcellular Localization
The subcellular localization of this compound is likely to be determined by the cellular machinery involved in oligodeoxyribonucleotide synthesis It may be directed to specific compartments or organelles based on the requirements of this process
Preparation Methods
Synthetic Routes and Reaction Conditions
The synthesis of N6-Methyl-dA phosphoramidite typically involves a multi-step process starting from inosine. One of the improved methods includes a five-step synthesis utilizing a 1-H-benzotriazol-1-yloxytris(dimethylamino)phosphoniumhexafluorophosphate (BOP)-mediated nucleophilic aromatic substitution (SNAr) reaction . The key steps involve:
- Protection of hydroxyl groups.
- Vilsmeier-type aryl chlorination.
- Reaction with methylamine.
- Cleavage of protecting groups.
- Phosphoramidite protection.
Industrial Production Methods
Industrial production of N6-Methyl-dA phosphoramidite involves scaling up the laboratory synthesis methods. The process is optimized for higher yields and purity, often involving automated synthesis and stringent quality control measures to ensure consistency and traceability .
Chemical Reactions Analysis
Types of Reactions
N6-Methyl-dA phosphoramidite undergoes various chemical reactions, including:
Substitution Reactions: The SNAr reaction is a key step in its synthesis.
Oxidation and Reduction: These reactions are less common but can be used to modify the compound further.
Common Reagents and Conditions
BOP Reagent: Used in the SNAr reaction for efficient synthesis.
Methylamine: Reacts with the chlorinated intermediate to introduce the methyl group.
Major Products
The primary product of these reactions is N6-Methyl-dA phosphoramidite, which can be further used in oligonucleotide synthesis .
Scientific Research Applications
N6-Methyl-dA phosphoramidite has a wide range of applications in scientific research:
Chemistry: Used in the synthesis of modified oligonucleotides for studying DNA methylation.
Biology: Helps in understanding the role of DNA methylation in gene expression and regulation.
Medicine: Potential applications in developing therapeutic oligonucleotides for treating genetic disorders.
Industry: Used in the production of diagnostic assays and molecular probes.
Comparison with Similar Compounds
Similar Compounds
N6-Methyladenosine: Another methylated nucleoside used in RNA studies.
N6-Ethyladenosine: Similar to N6-Methyl-dA but with an ethyl group instead of a methyl group.
Uniqueness
N6-Methyl-dA phosphoramidite is unique due to its specific application in DNA synthesis and its ability to mimic natural DNA methylation. This makes it a valuable tool in epigenetic research and the development of therapeutic oligonucleotides .
Properties
IUPAC Name |
3-[[(2R,3S,5R)-2-[[bis(4-methoxyphenyl)-phenylmethoxy]methyl]-5-[6-(methylamino)purin-9-yl]oxolan-3-yl]oxy-[di(propan-2-yl)amino]phosphanyl]oxypropanenitrile | |
---|---|---|
Details | Computed by Lexichem TK 2.7.0 (PubChem release 2021.05.07) | |
Source | PubChem | |
URL | https://pubchem.ncbi.nlm.nih.gov | |
Description | Data deposited in or computed by PubChem | |
InChI |
InChI=1S/C41H50N7O6P/c1-28(2)48(29(3)4)55(52-23-11-22-42)54-35-24-37(47-27-46-38-39(43-5)44-26-45-40(38)47)53-36(35)25-51-41(30-12-9-8-10-13-30,31-14-18-33(49-6)19-15-31)32-16-20-34(50-7)21-17-32/h8-10,12-21,26-29,35-37H,11,23-25H2,1-7H3,(H,43,44,45)/t35-,36+,37+,55?/m0/s1 | |
Details | Computed by InChI 1.0.6 (PubChem release 2021.05.07) | |
Source | PubChem | |
URL | https://pubchem.ncbi.nlm.nih.gov | |
Description | Data deposited in or computed by PubChem | |
InChI Key |
PTWRBAYAPDVZGR-JNTXQERPSA-N | |
Details | Computed by InChI 1.0.6 (PubChem release 2021.05.07) | |
Source | PubChem | |
URL | https://pubchem.ncbi.nlm.nih.gov | |
Description | Data deposited in or computed by PubChem | |
Canonical SMILES |
CC(C)N(C(C)C)P(OCCC#N)OC1CC(OC1COC(C2=CC=CC=C2)(C3=CC=C(C=C3)OC)C4=CC=C(C=C4)OC)N5C=NC6=C(N=CN=C65)NC | |
Details | Computed by OEChem 2.3.0 (PubChem release 2021.05.07) | |
Source | PubChem | |
URL | https://pubchem.ncbi.nlm.nih.gov | |
Description | Data deposited in or computed by PubChem | |
Isomeric SMILES |
CC(C)N(C(C)C)P(OCCC#N)O[C@H]1C[C@@H](O[C@@H]1COC(C2=CC=CC=C2)(C3=CC=C(C=C3)OC)C4=CC=C(C=C4)OC)N5C=NC6=C(N=CN=C65)NC | |
Details | Computed by OEChem 2.3.0 (PubChem release 2021.05.07) | |
Source | PubChem | |
URL | https://pubchem.ncbi.nlm.nih.gov | |
Description | Data deposited in or computed by PubChem | |
Molecular Formula |
C41H50N7O6P | |
Details | Computed by PubChem 2.1 (PubChem release 2021.05.07) | |
Source | PubChem | |
URL | https://pubchem.ncbi.nlm.nih.gov | |
Description | Data deposited in or computed by PubChem | |
Molecular Weight |
767.9 g/mol | |
Details | Computed by PubChem 2.1 (PubChem release 2021.05.07) | |
Source | PubChem | |
URL | https://pubchem.ncbi.nlm.nih.gov | |
Description | Data deposited in or computed by PubChem | |
Retrosynthesis Analysis
AI-Powered Synthesis Planning: Our tool employs the Template_relevance Pistachio, Template_relevance Bkms_metabolic, Template_relevance Pistachio_ringbreaker, Template_relevance Reaxys, Template_relevance Reaxys_biocatalysis model, leveraging a vast database of chemical reactions to predict feasible synthetic routes.
One-Step Synthesis Focus: Specifically designed for one-step synthesis, it provides concise and direct routes for your target compounds, streamlining the synthesis process.
Accurate Predictions: Utilizing the extensive PISTACHIO, BKMS_METABOLIC, PISTACHIO_RINGBREAKER, REAXYS, REAXYS_BIOCATALYSIS database, our tool offers high-accuracy predictions, reflecting the latest in chemical research and data.
Strategy Settings
Precursor scoring | Relevance Heuristic |
---|---|
Min. plausibility | 0.01 |
Model | Template_relevance |
Template Set | Pistachio/Bkms_metabolic/Pistachio_ringbreaker/Reaxys/Reaxys_biocatalysis |
Top-N result to add to graph | 6 |
Feasible Synthetic Routes
Disclaimer and Information on In-Vitro Research Products
Please be aware that all articles and product information presented on BenchChem are intended solely for informational purposes. The products available for purchase on BenchChem are specifically designed for in-vitro studies, which are conducted outside of living organisms. In-vitro studies, derived from the Latin term "in glass," involve experiments performed in controlled laboratory settings using cells or tissues. It is important to note that these products are not categorized as medicines or drugs, and they have not received approval from the FDA for the prevention, treatment, or cure of any medical condition, ailment, or disease. We must emphasize that any form of bodily introduction of these products into humans or animals is strictly prohibited by law. It is essential to adhere to these guidelines to ensure compliance with legal and ethical standards in research and experimentation.