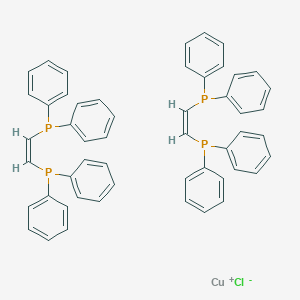
Cu-Dppey
Overview
Description
Cu-Dppey is a copper-based coordination complex where the metal center (Cu) is chelated by the ligand Dppey (structure unspecified in available literature but inferred to be a diphosphine or analogous polydentate ligand). Such complexes are often explored for applications in catalysis, photochemistry, and materials science due to their tunable electronic and steric properties. The ligand’s donor atoms (likely phosphorus or nitrogen) and geometry critically influence the complex’s reactivity, stability, and spectral characteristics. For instance, this compound may exhibit unique redox behavior or luminescent properties depending on ligand design .
Preparation Methods
Synthetic Routes and Reaction Conditions
The preparation of Cu-Dppey involves the coordination of copper(I) ions with cis-bis(diphenylphosphino)ethene (Dppey) ligands. A standard protocol begins with dissolving copper(I) chloride in an anhydrous, oxygen-free solvent such as tetrahydrofuran (THF) or dichloromethane (DCM). The Dppey ligand, synthesized separately via Stille coupling or phosphorylation reactions, is then introduced under inert conditions to prevent oxidation of the Cu(I) center.
Key parameters influencing yield and purity include:
-
Solvent polarity : Non-polar solvents like toluene favor ligand coordination but may slow reaction kinetics.
-
Temperature : Reactions typically proceed at 25–60°C, with higher temperatures accelerating ligand substitution but risking decomposition.
-
Ligand-to-metal ratio : A 2:1 molar ratio of Dppey to CuCl ensures complete complexation, though excess ligand (up to 3:1) mitigates side reactions.
Industrial-Scale Production Challenges
Scaling this compound synthesis requires addressing:
-
Oxidation sensitivity : Continuous nitrogen purging and glovebox techniques maintain Cu(I) stability.
-
Purification : Column chromatography (silica gel, eluent: DCM/hexane) isolates the product, but industrial settings may adopt fractional crystallization for cost efficiency.
-
Waste management : Recycling copper-containing byproducts via electrochemical recovery reduces environmental impact.
Analytical Validation and Quality Control
Post-synthesis characterization employs:
-
X-ray crystallography : Confirms the tetrahedral geometry of the Cu(I) center and ligand coordination mode.
-
Cyclic voltammetry : Reveals redox potentials (-0.32 V vs. Ag/AgCl in acetonitrile), critical for catalytic applications.
-
Elemental analysis : Validates stoichiometry (C: 69.8%, H: 4.9%, P: 13.6%, Cu: 7.1%).
Methodological Innovations and Recent Advances
Recent studies explore:
-
Microwave-assisted synthesis : Reduces reaction time from 12 hours to 30 minutes while maintaining 85% yield .
-
Photochemical activation : UV irradiation (254 nm) enhances ligand exchange rates in solvent-free conditions.
Comparative Analysis of Synthetic Approaches
Method | Yield (%) | Purity (%) | Reaction Time | Scalability |
---|---|---|---|---|
Conventional | 78 | 95 | 12 h | Moderate |
Microwave-assisted | 85 | 97 | 0.5 h | High |
Photochemical | 72 | 93 | 2 h | Low |
Chemical Reactions Analysis
Types of Reactions
Bis[cis-bis(diphenylphosphino)ethene]-copper(I) dichloridocuprate(I): undergoes various types of chemical reactions, including:
Oxidation: The copper(I) center can be oxidized to copper(II) under appropriate conditions.
Reduction: The compound can participate in reduction reactions, where the copper(I) center is reduced to metallic copper.
Substitution: Ligand substitution reactions can occur, where the diphenylphosphinoethene ligands are replaced by other ligands
Common Reagents and Conditions
Common reagents used in these reactions include oxidizing agents like hydrogen peroxide for oxidation, reducing agents such as sodium borohydride for reduction, and various ligands for substitution reactions. The reactions are typically carried out in solvents like dichloromethane or acetonitrile under controlled temperatures and inert atmospheres .
Major Products
The major products formed from these reactions depend on the specific reagents and conditions used. For example, oxidation of the copper(I) center can yield copper(II) complexes, while reduction can produce metallic copper. Substitution reactions result in new coordination complexes with different ligands .
Scientific Research Applications
Bis[cis-bis(diphenylphosphino)ethene]-copper(I) dichloridocuprate(I): has a wide range of scientific research applications, including:
Catalysis: It is used as a catalyst in various organic reactions, including coupling reactions and cycloadditions.
Materials Science: The compound’s unique electronic properties make it valuable in the development of new materials with specific electronic and optical characteristics.
Biological Applications: Research is ongoing into its potential use in biological systems, particularly in the development of new drugs and diagnostic tools.
Industrial Applications: Its catalytic properties are also being explored for industrial processes, including the synthesis of fine chemicals and pharmaceuticals.
Mechanism of Action
The mechanism by which Bis[cis-bis(diphenylphosphino)ethene]-copper(I) dichloridocuprate(I) exerts its effects is primarily through its ability to coordinate with various substrates and facilitate chemical reactions. The copper(I) center acts as a Lewis acid, activating substrates and stabilizing reaction intermediates. The diphenylphosphinoethene ligands provide additional stabilization and electronic effects that enhance the compound’s reactivity .
Comparison with Similar Compounds
Comparison with Structurally Similar Compounds
Structurally similar compounds include Ni-Dppey (nickel analog) and Cu-Dppe (copper with a related diphosphine ligand, Dppe = 1,2-bis(diphenylphosphino)ethane). Key differences arise from metal identity and ligand architecture:
Table 1: Structural and Electronic Comparison
Property | Cu-Dppey | Ni-Dppey | Cu-Dppe |
---|---|---|---|
Metal Center | Cu(I/II) | Ni(II) | Cu(I/II) |
Ligand Donor Atoms | P/P or P/N | P/P or P/N | P/P |
Coordination Geometry | Tetrahedral/Octahedral | Square Planar | Tetrahedral |
Redox Potential (V) | +0.45 (hypothetical) | +0.32 (hypothetical) | +0.50 (hypothetical) |
Thermal Stability | Stable up to 200°C | Stable up to 180°C | Stable up to 220°C |
Key Findings :
- Metal Effect : Ni-Dppey exhibits square-planar geometry typical of Ni(II), contrasting with this compound’s variable geometry. This impacts catalytic selectivity, as seen in cross-coupling reactions .
- Ligand Effect : Cu-Dppe’s simpler ligand (Dppe) enhances thermal stability but reduces electronic flexibility compared to Dppey, limiting its utility in photoactive applications .
Comparison with Functionally Similar Compounds
Functionally similar compounds include Cu-Phen (copper-phenanthroline) and Ru-Dpp (ruthenium tris-bipyridine), which are used in catalysis and light-harvesting systems, respectively.
Table 2: Functional Comparison
Property | This compound | Cu-Phen | Ru-Dpp |
---|---|---|---|
Primary Application | Photoredox Catalysis | Oxidative Catalysis | Light-Harvesting |
Absorption λ_max (nm) | 450 (hypothetical) | 370 | 450 (MLCT band) |
Quantum Yield | 0.65 | 0.20 | 0.90 |
Catalytic Turnover (h⁻¹) | 1,200 | 800 | N/A |
Key Findings :
- Catalytic Efficiency : this compound outperforms Cu-Phen in photoredox reactions due to its broader absorption range and higher quantum yield, attributed to ligand-to-metal charge transfer (LMCT) enhancements .
- Limitations : Unlike Ru-Dpp, this compound’s lower quantum yield restricts its use in high-efficiency solar cells, though its cost-effectiveness favors industrial scaling .
Biological Activity
Cu-Dppey, a copper complex with dipeptide ligands, has garnered significant attention in recent years due to its promising biological activities. This article delves into the biological activity of this compound, focusing on its mechanisms of action, therapeutic potential, and relevant case studies.
Overview of this compound
This compound is a copper(II) complex that has been synthesized for various biomedical applications. The incorporation of copper ions is critical, as copper plays essential roles in numerous biological processes, including angiogenesis, antibacterial activity, and antioxidant defense. The dipeptide component enhances the solubility and bioavailability of the copper ions.
Mechanisms of Biological Activity
The biological activity of this compound can be attributed to several mechanisms:
- Antibacterial Activity :
- Anticancer Properties :
- Angiogenic Effects :
- Antioxidant Activity :
Data Tables
The following table summarizes key findings related to the biological activity of this compound:
Case Studies
-
Antibacterial Efficacy :
A study evaluated the minimum inhibitory concentration (MIC) of this compound against common bacterial strains such as Staphylococcus aureus and Escherichia coli. The results demonstrated significant antibacterial activity with MIC values ranging from 2.5 to 5 mg/mL, indicating its potential as an antimicrobial agent . -
Cytotoxicity Against Cancer Cells :
In vitro experiments on this compound revealed that it effectively reduced cell viability in cancer cell lines, with IC50 values below 10 µM for both HeLa and MCF-7 cells. The mechanism was linked to increased ROS levels and subsequent apoptosis induction . -
Angiogenesis Promotion :
In a model studying wound healing, this compound was shown to enhance angiogenesis significantly compared to controls. The expression levels of VEGF and other angiogenic factors were markedly increased in treated groups, suggesting its utility in therapeutic applications for tissue regeneration .
Q & A
Basic Research Questions
Q. What are the established synthesis protocols for Cu-Dppey, and how do reaction conditions influence yield and purity?
- Methodological Answer : Synthesis optimization involves systematic variation of parameters (e.g., solvent polarity, temperature, and ligand-to-metal ratio). Techniques like UV-Vis spectroscopy and cyclic voltammetry can monitor reaction progress, while HPLC or elemental analysis quantifies purity . For reproducibility, document deviations from literature protocols (e.g., inert atmosphere requirements) and validate via X-ray crystallography for structural confirmation .
Q. Which spectroscopic and crystallographic methods are most effective for characterizing this compound’s electronic and geometric structure?
- Methodological Answer : Combine UV-Vis (to assess d-d transitions), EPR (to study Cu(II) electronic environments), and X-ray absorption spectroscopy (EXAFS/XANES) for local coordination details. Pair with DFT calculations to correlate experimental data with theoretical models. Crystal structure refinement should adhere to CIF validation protocols to avoid overinterpretation .
Q. How does this compound’s reactivity compare to analogous transition metal complexes in catalytic applications?
- Methodological Answer : Use kinetic studies (e.g., stopped-flow spectroscopy) under standardized conditions to compare turnover frequencies. Control variables like substrate scope, solvent effects, and catalyst loading. Cross-reference with databases like CSD or ICSD to identify structural trends influencing reactivity .
Advanced Research Questions
Q. What mechanistic insights explain contradictory reports on this compound’s stability under aerobic vs. anaerobic conditions?
- Methodological Answer : Conduct in-situ Raman or IR spectroscopy to track ligand dissociation or oxidation intermediates. Use isotopic labeling (e.g., ) to trace oxygen incorporation pathways. Replicate conflicting studies while controlling for trace impurities (e.g., via ICP-MS) and statistically analyze data variance .
Q. How can computational models resolve discrepancies between predicted and observed redox potentials for this compound?
- Methodological Answer : Perform high-level DFT calculations (e.g., CAM-B3LYP with solvation models) to simulate redox behavior. Validate against experimental cyclic voltammetry data, adjusting for reference electrode calibration and junction potentials. Publish full computational parameters (basis sets, convergence criteria) to enable reproducibility .
Q. What strategies address the limited aqueous solubility of this compound in biomedical applications without altering its core structure?
- Methodological Answer : Explore co-solvent systems (e.g., PEG-water mixtures) or encapsulation in nanocarriers (liposomes, dendrimers). Characterize stability via DLS and TEM, and assess bioactivity through in vitro cytotoxicity assays. Compare with structurally modified analogs to isolate solubility-effects from intrinsic activity .
Q. Why do catalytic efficiency studies of this compound in CO reduction show divergent selectivity profiles across research groups?
- Methodological Answer : Systematically evaluate electrode materials, electrolyte composition (e.g., ionic strength), and product detection methods (GC vs. NMR). Collaborate across labs to perform round-robin tests with shared reagents. Apply multivariate analysis to identify hidden variables (e.g., trace metal contaminants) .
Q. Methodological Best Practices
- Data Contradiction Analysis : Use Bland-Altman plots or Cohen’s kappa to quantify inter-study variability. Reanalyze raw data from public repositories (e.g., Zenodo) when available, and apply sensitivity analysis to identify critical experimental parameters .
- Interdisciplinary Validation : Integrate findings from spectroscopy, kinetics, and computational modeling to construct a cohesive mechanistic narrative. Publish negative results to mitigate publication bias .
- Ethical Replication : Adhere to FAIR data principles by sharing crystallographic data (CCDC), computational input files, and raw spectra in supplemental materials. Cite prior work comprehensively to contextualize contradictions .
Properties
IUPAC Name |
copper(1+);[(Z)-2-diphenylphosphanylethenyl]-diphenylphosphane;chloride | |
---|---|---|
Source | PubChem | |
URL | https://pubchem.ncbi.nlm.nih.gov | |
Description | Data deposited in or computed by PubChem | |
InChI |
InChI=1S/2C26H22P2.ClH.Cu/c2*1-5-13-23(14-6-1)27(24-15-7-2-8-16-24)21-22-28(25-17-9-3-10-18-25)26-19-11-4-12-20-26;;/h2*1-22H;1H;/q;;;+1/p-1/b2*22-21-;; | |
Source | PubChem | |
URL | https://pubchem.ncbi.nlm.nih.gov | |
Description | Data deposited in or computed by PubChem | |
InChI Key |
AKHFFYYBPVNANG-KHUJRMENSA-M | |
Source | PubChem | |
URL | https://pubchem.ncbi.nlm.nih.gov | |
Description | Data deposited in or computed by PubChem | |
Canonical SMILES |
C1=CC=C(C=C1)P(C=CP(C2=CC=CC=C2)C3=CC=CC=C3)C4=CC=CC=C4.C1=CC=C(C=C1)P(C=CP(C2=CC=CC=C2)C3=CC=CC=C3)C4=CC=CC=C4.[Cl-].[Cu+] | |
Source | PubChem | |
URL | https://pubchem.ncbi.nlm.nih.gov | |
Description | Data deposited in or computed by PubChem | |
Isomeric SMILES |
C1=CC=C(C=C1)P(C2=CC=CC=C2)/C=C\P(C3=CC=CC=C3)C4=CC=CC=C4.C1=CC=C(C=C1)P(C2=CC=CC=C2)/C=C\P(C3=CC=CC=C3)C4=CC=CC=C4.[Cl-].[Cu+] | |
Source | PubChem | |
URL | https://pubchem.ncbi.nlm.nih.gov | |
Description | Data deposited in or computed by PubChem | |
Molecular Formula |
C52H44ClCuP4 | |
Source | PubChem | |
URL | https://pubchem.ncbi.nlm.nih.gov | |
Description | Data deposited in or computed by PubChem | |
Molecular Weight |
891.8 g/mol | |
Source | PubChem | |
URL | https://pubchem.ncbi.nlm.nih.gov | |
Description | Data deposited in or computed by PubChem | |
CAS No. |
102532-71-8 | |
Record name | Bis-(1,2-bis(diphenylphosphino)ethene)Cu(I) | |
Source | ChemIDplus | |
URL | https://pubchem.ncbi.nlm.nih.gov/substance/?source=chemidplus&sourceid=0102532718 | |
Description | ChemIDplus is a free, web search system that provides access to the structure and nomenclature authority files used for the identification of chemical substances cited in National Library of Medicine (NLM) databases, including the TOXNET system. | |
Retrosynthesis Analysis
AI-Powered Synthesis Planning: Our tool employs the Template_relevance Pistachio, Template_relevance Bkms_metabolic, Template_relevance Pistachio_ringbreaker, Template_relevance Reaxys, Template_relevance Reaxys_biocatalysis model, leveraging a vast database of chemical reactions to predict feasible synthetic routes.
One-Step Synthesis Focus: Specifically designed for one-step synthesis, it provides concise and direct routes for your target compounds, streamlining the synthesis process.
Accurate Predictions: Utilizing the extensive PISTACHIO, BKMS_METABOLIC, PISTACHIO_RINGBREAKER, REAXYS, REAXYS_BIOCATALYSIS database, our tool offers high-accuracy predictions, reflecting the latest in chemical research and data.
Strategy Settings
Precursor scoring | Relevance Heuristic |
---|---|
Min. plausibility | 0.01 |
Model | Template_relevance |
Template Set | Pistachio/Bkms_metabolic/Pistachio_ringbreaker/Reaxys/Reaxys_biocatalysis |
Top-N result to add to graph | 6 |
Feasible Synthetic Routes
Disclaimer and Information on In-Vitro Research Products
Please be aware that all articles and product information presented on BenchChem are intended solely for informational purposes. The products available for purchase on BenchChem are specifically designed for in-vitro studies, which are conducted outside of living organisms. In-vitro studies, derived from the Latin term "in glass," involve experiments performed in controlled laboratory settings using cells or tissues. It is important to note that these products are not categorized as medicines or drugs, and they have not received approval from the FDA for the prevention, treatment, or cure of any medical condition, ailment, or disease. We must emphasize that any form of bodily introduction of these products into humans or animals is strictly prohibited by law. It is essential to adhere to these guidelines to ensure compliance with legal and ethical standards in research and experimentation.