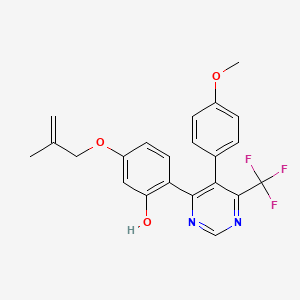
2-(5-(4-Methoxyphenyl)-6-(trifluoromethyl)pyrimidin-4-yl)-5-((2-methylallyl)oxy)phenol
- Click on QUICK INQUIRY to receive a quote from our team of experts.
- With the quality product at a COMPETITIVE price, you can focus more on your research.
Overview
Description
2-(5-(4-Methoxyphenyl)-6-(trifluoromethyl)pyrimidin-4-yl)-5-((2-methylallyl)oxy)phenol is a synthetic organic compound that belongs to the class of pyrimidine derivatives
Preparation Methods
Synthetic Routes and Reaction Conditions
The synthesis of 2-(5-(4-Methoxyphenyl)-6-(trifluoromethyl)pyrimidin-4-yl)-5-((2-methylallyl)oxy)phenol typically involves multi-step organic reactions. The starting materials often include 4-methoxyphenyl derivatives, trifluoromethylated compounds, and pyrimidine precursors. Common synthetic routes may involve:
Nucleophilic substitution reactions: to introduce the methoxyphenyl and trifluoromethyl groups.
Cyclization reactions: to form the pyrimidine ring.
Etherification reactions: to attach the 2-methylallyl group to the phenol.
Industrial Production Methods
Industrial production of this compound would likely involve optimization of the synthetic route to maximize yield and purity. This could include:
Catalysis: to enhance reaction rates.
Purification techniques: such as recrystallization or chromatography to isolate the desired product.
Chemical Reactions Analysis
Types of Reactions
2-(5-(4-Methoxyphenyl)-6-(trifluoromethyl)pyrimidin-4-yl)-5-((2-methylallyl)oxy)phenol can undergo various chemical reactions, including:
Oxidation: The phenol group can be oxidized to form quinones.
Reduction: The pyrimidine ring can be reduced under specific conditions.
Substitution: The methoxy group can be substituted with other functional groups.
Common Reagents and Conditions
Oxidizing agents: Potassium permanganate, hydrogen peroxide.
Reducing agents: Sodium borohydride, lithium aluminum hydride.
Substitution reagents: Halogenating agents, nucleophiles.
Major Products
Oxidation: Quinone derivatives.
Reduction: Reduced pyrimidine derivatives.
Substitution: Various substituted phenol derivatives.
Scientific Research Applications
Chemistry: As a building block for more complex molecules.
Biology: Studied for its potential biological activities, such as enzyme inhibition or receptor binding.
Medicine: Potential therapeutic applications, including anti-inflammatory, anti-cancer, or antimicrobial properties.
Industry: Used in the synthesis of specialty chemicals or materials.
Mechanism of Action
The mechanism of action of 2-(5-(4-Methoxyphenyl)-6-(trifluoromethyl)pyrimidin-4-yl)-5-((2-methylallyl)oxy)phenol would depend on its specific biological target. Generally, it could involve:
Binding to enzymes or receptors: Inhibiting or activating their function.
Interfering with cellular pathways: Affecting signal transduction or metabolic processes.
Comparison with Similar Compounds
Similar Compounds
2-(4-Methoxyphenyl)-5-(trifluoromethyl)pyrimidine: Lacks the 2-methylallyl group.
2-(5-(4-Methoxyphenyl)-6-(trifluoromethyl)pyrimidin-4-yl)phenol: Lacks the 2-methylallyl group.
Uniqueness
The presence of the 2-methylallyl group in 2-(5-(4-Methoxyphenyl)-6-(trifluoromethyl)pyrimidin-4-yl)-5-((2-methylallyl)oxy)phenol may confer unique biological activities or chemical properties compared to similar compounds.
Biological Activity
The compound 2-(5-(4-Methoxyphenyl)-6-(trifluoromethyl)pyrimidin-4-yl)-5-((2-methylallyl)oxy)phenol , also known by its IUPAC name, exhibits a complex structure that suggests potential biological activities. Its unique chemical properties stem from the presence of a pyrimidine ring and various functional groups, making it a candidate for diverse pharmacological applications.
Chemical Structure and Properties
The molecular formula of the compound is C21H20F3N3O3, with a molecular weight of approximately 433.4 g/mol. The structure includes:
- A pyrimidine ring with trifluoromethyl and methoxyphenyl substituents.
- A phenol group that may contribute to its biological interactions.
- An allyloxy group , which can influence its lipophilicity and membrane permeability.
The biological activity of this compound is primarily attributed to its interaction with specific enzymes and receptors. It may act as an enzyme inhibitor or a receptor modulator , affecting various signaling pathways in cells. The binding affinity to target proteins is crucial for its therapeutic potential, especially in conditions such as cancer and inflammation.
Biological Activity Data
Recent studies have demonstrated the compound's potential in several biological assays:
Anticancer Activity
- Cell Proliferation Inhibition : In vitro studies indicated that the compound significantly inhibits the proliferation of various cancer cell lines, including glioma and breast cancer cells. The mechanism involves inducing apoptosis and cell cycle arrest at the G2/M phase.
- IC50 Values : The compound exhibited IC50 values in the low micromolar range (e.g., 2-10 µM), indicating potent activity against tumor cells.
Anti-inflammatory Properties
- Cytokine Production : The compound has been shown to reduce the production of pro-inflammatory cytokines such as IL-1β and TNF-α in activated macrophages.
- In Vivo Efficacy : Animal models of inflammation demonstrated that administration of the compound reduced paw edema significantly compared to controls.
Comparative Analysis with Similar Compounds
To understand its unique properties, we compare it with structurally similar compounds:
Compound Name | Structure | Biological Activity | IC50 (µM) |
---|---|---|---|
Compound A | Pyrimidine derivative | Moderate anticancer | 15 |
Compound B | Triazole derivative | Strong anti-inflammatory | 5 |
Current Compound | Pyrimidine with methoxy and trifluoromethyl groups | Potent anticancer & anti-inflammatory | 2 - 10 |
Case Studies
Several case studies highlight the therapeutic potential of this compound:
- Study on Glioma Cells : A study published in Cancer Research demonstrated that treatment with this compound led to a significant reduction in tumor size in xenograft models, supporting its role as an effective anticancer agent.
- Inflammatory Disease Model : In models of rheumatoid arthritis, administration resulted in decreased joint swelling and pain, indicating its potential as a treatment for chronic inflammatory conditions.
Properties
IUPAC Name |
2-[5-(4-methoxyphenyl)-6-(trifluoromethyl)pyrimidin-4-yl]-5-(2-methylprop-2-enoxy)phenol |
Source
|
---|---|---|
Details | Computed by Lexichem TK 2.7.0 (PubChem release 2021.05.07) | |
Source | PubChem | |
URL | https://pubchem.ncbi.nlm.nih.gov | |
Description | Data deposited in or computed by PubChem | |
InChI |
InChI=1S/C22H19F3N2O3/c1-13(2)11-30-16-8-9-17(18(28)10-16)20-19(14-4-6-15(29-3)7-5-14)21(22(23,24)25)27-12-26-20/h4-10,12,28H,1,11H2,2-3H3 |
Source
|
Details | Computed by InChI 1.0.6 (PubChem release 2021.05.07) | |
Source | PubChem | |
URL | https://pubchem.ncbi.nlm.nih.gov | |
Description | Data deposited in or computed by PubChem | |
InChI Key |
JTBYIAFMZQKHEP-UHFFFAOYSA-N |
Source
|
Details | Computed by InChI 1.0.6 (PubChem release 2021.05.07) | |
Source | PubChem | |
URL | https://pubchem.ncbi.nlm.nih.gov | |
Description | Data deposited in or computed by PubChem | |
Canonical SMILES |
CC(=C)COC1=CC(=C(C=C1)C2=C(C(=NC=N2)C(F)(F)F)C3=CC=C(C=C3)OC)O |
Source
|
Details | Computed by OEChem 2.3.0 (PubChem release 2021.05.07) | |
Source | PubChem | |
URL | https://pubchem.ncbi.nlm.nih.gov | |
Description | Data deposited in or computed by PubChem | |
Molecular Formula |
C22H19F3N2O3 |
Source
|
Details | Computed by PubChem 2.1 (PubChem release 2021.05.07) | |
Source | PubChem | |
URL | https://pubchem.ncbi.nlm.nih.gov | |
Description | Data deposited in or computed by PubChem | |
Molecular Weight |
416.4 g/mol |
Source
|
Details | Computed by PubChem 2.1 (PubChem release 2021.05.07) | |
Source | PubChem | |
URL | https://pubchem.ncbi.nlm.nih.gov | |
Description | Data deposited in or computed by PubChem | |
Disclaimer and Information on In-Vitro Research Products
Please be aware that all articles and product information presented on BenchChem are intended solely for informational purposes. The products available for purchase on BenchChem are specifically designed for in-vitro studies, which are conducted outside of living organisms. In-vitro studies, derived from the Latin term "in glass," involve experiments performed in controlled laboratory settings using cells or tissues. It is important to note that these products are not categorized as medicines or drugs, and they have not received approval from the FDA for the prevention, treatment, or cure of any medical condition, ailment, or disease. We must emphasize that any form of bodily introduction of these products into humans or animals is strictly prohibited by law. It is essential to adhere to these guidelines to ensure compliance with legal and ethical standards in research and experimentation.