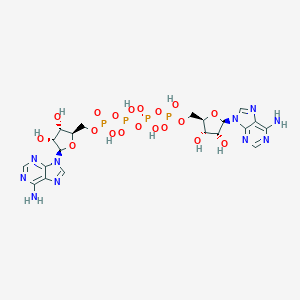
Diadenosine tetraphosphate
Overview
Description
Diadenosine tetraphosphate (Ap4A) is a dinucleoside polyphosphate comprising two adenosine moieties linked by four phosphate groups. First synthesized enzymatically by lysyl-sRNA synthetase in 1966 , Ap4A has since been identified as a multifunctional molecule with roles in stress signaling, nucleotide metabolism, and cellular regulation. In bacteria, it acts as a secondary messenger, influencing stress responses and gene expression . In eukaryotes, Ap4A modulates platelet aggregation, vasoconstriction, and DNA repair . Its intracellular levels are tightly regulated by hydrolases such as ApaH, which cleaves Ap4A into two ADP molecules .
Preparation Methods
Synthetic Routes and Reaction Conditions: Diadenosine tetraphosphate can be synthesized enzymatically or chemically. Enzymatic synthesis often involves aminoacyl-tRNA synthetases, DNA and RNA ligases, and ubiquitin-activating enzymes. These enzymes facilitate the formation of this compound through the transfer of adenosine monophosphate to adenosine triphosphate .
Industrial Production Methods: Industrial production of this compound typically involves large-scale enzymatic reactions using purified enzymes. The reaction conditions include optimal pH, temperature, and the presence of necessary cofactors such as magnesium ions. High-performance liquid chromatography (HPLC) is commonly used to purify the product .
Chemical Reactions Analysis
Types of Reactions: Diadenosine tetraphosphate undergoes various chemical reactions, including hydrolysis, phosphorylation, and binding interactions with proteins. It is hydrolyzed by specific hydrolases such as ApaH, which breaks down the compound into adenosine and adenosine triphosphate .
Common Reagents and Conditions: Common reagents used in reactions involving this compound include nucleotides, metal ions (e.g., magnesium), and specific enzymes like adenylate kinase. The reactions typically occur under physiological conditions, such as neutral pH and moderate temperatures .
Major Products: The major products formed from the hydrolysis of this compound are adenosine and adenosine triphosphate. Other reactions may produce various phosphorylated intermediates depending on the specific enzymes and conditions used .
Scientific Research Applications
Cellular Signaling and Stress Response
Ap4A acts as a second messenger in both prokaryotic and eukaryotic cells, influencing cellular responses to stress. It has been identified as an important alarmone , which triggers adaptive mechanisms during environmental challenges. Studies indicate that Ap4A concentrations increase significantly under stress conditions, suggesting its role in cellular survival strategies.
Case Study: Bacterial Stress Response
Research has shown that Ap4A is crucial for bacteria facing environmental stressors. It modulates cellular functions to enhance survivability, indicating its potential as a target for antimicrobial therapies .
Cardiovascular Applications
Ap4A has demonstrated significant effects on cardiovascular physiology. It can be released from activated platelets and has been studied for its impact on coronary arterial microvessels.
Case Study: Hypotensive Effects
In clinical studies involving healthy male volunteers, Ap4A administration resulted in a dose-dependent hypotensive effect, reducing mean arterial pressure by up to 60% compared to control values. This suggests its potential use in managing blood pressure during surgical procedures requiring controlled hypotension .
Neurological Applications
The protective effects of Ap4A have been explored in neurological contexts, particularly concerning neurodegenerative diseases.
Case Study: Stroke and Parkinson's Disease
In rodent models of stroke and Parkinson's disease, intracerebroventricular administration of Ap4A showed protective effects against neuronal injuries. This positions Ap4A as a promising therapeutic candidate for neuroprotection in degenerative conditions .
Ocular Health
Ap4A is present in ocular fluids and influences various ocular functions, including tear secretion and intraocular pressure regulation.
Research and Development
Ongoing research continues to uncover the molecular mechanisms underlying Ap4A's actions and its interactions with various proteins involved in metabolism and gene expression.
Chemical Proteomics Insights
Recent studies employing chemical proteomics have identified numerous protein interactors of Ap4A involved in fundamental cellular processes such as nucleotide metabolism and regulatory mechanisms .
Data Table: Summary of Applications
Mechanism of Action
Diadenosine tetraphosphate exerts its effects by acting as a secondary messenger in cellular signaling pathways. It binds to specific protein targets, such as adenylate kinase, and modulates their activity. This binding can influence various cellular processes, including gene expression, DNA repair, and immune responses. The compound’s role as an alarmone helps cells adapt to environmental and genotoxic stresses .
Comparison with Similar Compounds
Structural and Functional Analogues
Ap4A belongs to the dinucleoside polyphosphate (NpnN) family, which includes compounds like Ap3A, Ap5A, and diadenosine pentaphosphate (Ap5A). Key structural analogs and their biological roles are compared below:
Key Insights :
- Phosphate Group Impact : Increasing phosphate groups (e.g., Ap5A) enhances stability and receptor binding . Thiophosphate modifications (e.g., ApspCHClppsA) significantly boost antiplatelet potency .
Degradation and Hydrolase Specificity
Ap4A hydrolases (e.g., ApaH, YqeK) exhibit substrate specificity:
- ApaH: Cleaves Ap4A symmetrically into ADP; deletion in P. aeruginosa elevates Ap4A levels, suppressing 62.4% of genes .
- YqeK : Iron-dependent hydrolases that degrade Ap4A and regulate biofilm formation in diverse bacteria .
- DIPP Subfamily: Preferentially hydrolyzes diphosphoinositol polyphosphates over Ap4A .
Signaling and Stress Response
- Ap4A vs. aeruginosa but modulates transcriptional responses .
- Ap4A vs. c-di-GMP : While c-di-GMP drives biofilm formation, Ap4A influences motility and stress survival without affecting c-di-GMP homeostasis .
- Cross-Kingdom Roles : In eukaryotes, Ap4A and Ap5A act as neurotransmitters and vasoconstrictors, whereas bacterial Ap4A is linked to metabolic adaptation .
Clinical and Therapeutic Potential
Biological Activity
Diadenosine tetraphosphate (Ap4A) is a dinucleoside polyphosphate that plays a significant role in cellular signaling and metabolism across various organisms, including bacteria and eukaryotes. This article provides a comprehensive overview of the biological activity of Ap4A, highlighting its synthesis, degradation, physiological roles, and implications in disease states.
Overview of this compound
Ap4A is formed by the condensation of two adenosine monophosphates (AMP) with four phosphate groups. It is recognized as a second messenger that modulates various cellular processes in response to stress and other stimuli. Its concentration can dramatically increase under stress conditions, suggesting a role in cellular signaling pathways.
Synthesis and Degradation
The synthesis of Ap4A occurs through the action of specific enzymes, primarily This compound synthase , while its degradation is mediated by ApaH , an enzyme that hydrolyzes Ap4A into two ADP molecules. The regulation of Ap4A levels is crucial for maintaining cellular homeostasis, especially under stress conditions where its accumulation can trigger protective responses.
Enzyme | Function |
---|---|
This compound Synthase | Synthesizes Ap4A from AMP |
ApaH | Hydrolyzes Ap4A into ADP |
1. Cellular Stress Response
Ap4A has been implicated as an "alarmone," signaling the presence of stress within the cell. Increased levels of Ap4A have been observed in response to environmental stresses such as oxidative stress or genotoxic damage. For instance, studies show that Ap4A concentrations can rise significantly (up to 365 µM) following exposure to cadmium chloride (CdCl2), indicating its role in stress adaptation mechanisms .
2. Virulence Factor Regulation
In pathogenic bacteria like Pseudomonas aeruginosa, Ap4A plays a critical role in virulence. The enzyme ApaH, which degrades Ap4A, has been shown to be essential for the production of virulence factors. Loss of ApaH leads to increased levels of Ap4A, resulting in altered gene expression profiles that affect the bacterium's ability to cause infection .
3. Modulation of Cellular Metabolism
Ap4A influences various metabolic pathways by interacting with specific protein targets. It has been shown to affect tight junction proteins in corneal epithelial cells, enhancing the delivery efficiency of anti-glaucomatous drugs by modifying barrier functions . This highlights its potential therapeutic applications beyond its traditional roles in signaling.
Case Study 1: Pseudomonas aeruginosa
Research demonstrated that deletion of the apaH gene resulted in significant changes in gene expression—over 1,280 differentially regulated genes were identified, indicating that Ap4A accumulation has profound effects on bacterial physiology and virulence .
Case Study 2: Oxidative Stress Response
In experiments involving oxidative stress, cells treated with CdCl2 exhibited marked increases in Ap4A levels compared to control groups. This suggests that Ap4A acts as a mediator for cellular responses to oxidative damage, potentially influencing survival strategies during stress .
Q & A
Basic Research Questions
Q. What experimental approaches are used to detect and quantify Ap4A in biological systems?
Ap4A levels can be quantified using high-performance liquid chromatography (HPLC) with UV detection, as demonstrated in human tear analysis from Sjögren syndrome patients . For subpicomole sensitivity, a phosphodiesterase luciferin-luciferase coupled assay is employed, enabling specific detection of Ap4A hydrolase activity . In plant studies, nuclear localization of Ap4A hydrolase has been confirmed via subcellular fractionation combined with enzymatic assays .
Q. How is Ap4A synthesized in bacterial and eukaryotic systems?
Ap4A is primarily synthesized by aminoacyl-tRNA synthetases (e.g., seryl-tRNA and phenylalanyl-tRNA synthetases) under oxidative stress or metabolic perturbations. In Lupinus luteus and Euglena gracilis, these enzymes catalyze Ap4A formation during tRNA charging . In Salmonella typhimurium, propionate kinase (TdcD) also exhibits Ap4A synthetic activity when co-crystallized with ATP .
Q. What role does Ap4A play in bacterial physiology?
Ap4A acts as a second messenger regulating motility, biofilm formation, and antibiotic tolerance. In Pseudomonas aeruginosa, Ap4A accumulation (via apaH deletion) reduces motility but does not alter biofilm formation or c-di-GMP levels, highlighting species-specific regulatory roles . Ap4A also interacts with stress-response proteins (e.g., DnaK, GroEL), linking it to heat and oxidative stress adaptation .
Advanced Research Questions
Q. How does Ap4A influence bacterial virulence and antibiotic resistance mechanisms?
In P. aeruginosa, ApaH (an Ap4A hydrolase) deletion downregulates virulence genes (e.g., lasB, phzA1) and reduces infectivity in plant, insect, and mammalian models . Paradoxically, while apaH mutants show increased colistin resistance gene (eptA) expression, they exhibit no significant changes in antibiotic susceptibility to ciprofloxacin or aminoglycosides . Contrastingly, in Staphylococcus aureus, YqeK (Ap4A hydrolase) deletion enhances biofilm survival, suggesting Ap4A’s role in antibiotic evasion .
Q. What methodological strategies resolve contradictions in Ap4A’s role in stress responses?
Transcriptomic profiling (RNA-seq) of P. aeruginosa apaH mutants revealed 1,280 differentially expressed genes (DEGs), with 62.4% downregulated, indicating Ap4A’s suppressive effect on transcription . For S. aureus, RNA sequencing of yqeK mutants under biofilm conditions can clarify Ap4A’s regulatory network . In mammalian cells, stress-induced Ap4A accumulation (e.g., heat shock, ethanol) correlates with adaptive responses, measured via HPLC and stress-protein synthesis assays .
Q. How does Ap4A interact with eukaryotic signaling pathways?
Ap4A activates P2Y2 receptors in adrenal chromaffin cells, mobilizing intracellular Ca²⁺ stores . In cardiac tissue, Ap4A modulates action potential duration (APD95) under ischemic conditions, measured via electrophysiological recordings at varying pH levels . These effects are dose-dependent and reversible, suggesting therapeutic potential in ischemia-reperfusion injury models.
Q. What evidence links Ap4A dysregulation to human diseases?
Sjögren syndrome patients exhibit elevated tear Ap4A levels (up to 4.09 µM vs. 0.13 µM in controls), correlating with reduced Schirmer test scores . In cancer, the tumor suppressor FHIT regulates Ap4A metabolism; FHIT-deficient cells show altered diadenosine polyphosphate ratios, impacting apoptosis and proliferation .
Q. Methodological Considerations
Q. How to design experiments assessing Ap4A’s role in gene regulation?
- Bacterial systems : Combine apaH or yqeK knockout strains with RNA-seq to identify DEGs .
- Eukaryotic systems : Use siRNA knockdown of P2Y2 receptors or FHIT, paired with Ca²⁺ imaging or metabolomics .
- Stress models : Apply oxidative agents (e.g., H₂O₂) or heat shock, followed by Ap4A quantification via HPLC/mass spectrometry .
Q. How to address species-specific variability in Ap4A signaling?
Comparative studies across bacteria (P. aeruginosa, S. aureus) and eukaryotes (mammalian cells, plants) are critical. For example, apaH deletion reduces virulence in P. aeruginosa but enhances biofilm resilience in S. aureus, necessitating tailored genetic and phenotypic assays .
Q. Tables
Table 1. Ap4A Quantification Methods Across Studies
Method | Sensitivity | Application | Reference |
---|---|---|---|
HPLC-UV | µM range | Tear analysis (Sjögren syndrome) | |
Luciferase-coupled assay | Subpicomole | Enzyme activity assays | |
RNA-seq | Transcriptome-wide | Bacterial gene regulation |
Table 2. Ap4A-Related Enzymes and Their Roles
Enzyme | Organism | Function | Phenotype of Deletion |
---|---|---|---|
ApaH | P. aeruginosa | Ap4A hydrolysis | Reduced virulence, motility loss |
YqeK | S. aureus | Ap4A hydrolysis | Enhanced biofilm formation |
NudA | Helicobacter pylori | Ap4A metabolism | Impaired stress response |
Properties
CAS No. |
5542-28-9 |
---|---|
Molecular Formula |
C20H28N10O19P4 |
Molecular Weight |
836.4 g/mol |
IUPAC Name |
[[(2R,3S,4R,5R)-5-(6-aminopurin-9-yl)-3,4-dihydroxyoxolan-2-yl]methoxy-hydroxyphosphoryl] [[[(2R,3S,4R,5R)-5-(6-aminopurin-9-yl)-3,4-dihydroxyoxolan-2-yl]methoxy-hydroxyphosphoryl]oxy-hydroxyphosphoryl] hydrogen phosphate |
InChI |
InChI=1S/C20H28N10O19P4/c21-15-9-17(25-3-23-15)29(5-27-9)19-13(33)11(31)7(45-19)1-43-50(35,36)47-52(39,40)49-53(41,42)48-51(37,38)44-2-8-12(32)14(34)20(46-8)30-6-28-10-16(22)24-4-26-18(10)30/h3-8,11-14,19-20,31-34H,1-2H2,(H,35,36)(H,37,38)(H,39,40)(H,41,42)(H2,21,23,25)(H2,22,24,26)/t7-,8-,11-,12-,13-,14-,19-,20-/m1/s1 |
InChI Key |
YOAHKNVSNCMZGQ-XPWFQUROSA-N |
SMILES |
C1=NC(=C2C(=N1)N(C=N2)C3C(C(C(O3)COP(=O)(O)OP(=O)(O)OP(=O)(O)OP(=O)(O)OCC4C(C(C(O4)N5C=NC6=C(N=CN=C65)N)O)O)O)O)N |
Isomeric SMILES |
C1=NC(=C2C(=N1)N(C=N2)[C@H]3[C@@H]([C@@H]([C@H](O3)COP(=O)(O)OP(=O)(O)OP(=O)(O)OP(=O)(O)OC[C@@H]4[C@H]([C@H]([C@@H](O4)N5C=NC6=C(N=CN=C65)N)O)O)O)O)N |
Canonical SMILES |
C1=NC(=C2C(=N1)N(C=N2)C3C(C(C(O3)COP(=O)(O)OP(=O)(O)OP(=O)(O)OP(=O)(O)OCC4C(C(C(O4)N5C=NC6=C(N=CN=C65)N)O)O)O)O)N |
Key on ui other cas no. |
5542-28-9 |
physical_description |
Solid |
Synonyms |
[[[[(2R,3S,4R,5R)-5-(6-aminopurin-9-yl)-3,4-dihydroxyoxolan-2-yl]methoxy-hydroxyphosphoryl]oxy-hydroxyphosphoryl]oxy-hydroxyphosphoryl] [(2R,3S,4R,5R)-5-(6-aminopurin-9-yl)-3,4-dihydroxyoxolan-2-yl]methyl hydrogen phosphate; Diadenosine tetraphosphate |
Origin of Product |
United States |
Retrosynthesis Analysis
AI-Powered Synthesis Planning: Our tool employs the Template_relevance Pistachio, Template_relevance Bkms_metabolic, Template_relevance Pistachio_ringbreaker, Template_relevance Reaxys, Template_relevance Reaxys_biocatalysis model, leveraging a vast database of chemical reactions to predict feasible synthetic routes.
One-Step Synthesis Focus: Specifically designed for one-step synthesis, it provides concise and direct routes for your target compounds, streamlining the synthesis process.
Accurate Predictions: Utilizing the extensive PISTACHIO, BKMS_METABOLIC, PISTACHIO_RINGBREAKER, REAXYS, REAXYS_BIOCATALYSIS database, our tool offers high-accuracy predictions, reflecting the latest in chemical research and data.
Strategy Settings
Precursor scoring | Relevance Heuristic |
---|---|
Min. plausibility | 0.01 |
Model | Template_relevance |
Template Set | Pistachio/Bkms_metabolic/Pistachio_ringbreaker/Reaxys/Reaxys_biocatalysis |
Top-N result to add to graph | 6 |
Feasible Synthetic Routes
Disclaimer and Information on In-Vitro Research Products
Please be aware that all articles and product information presented on BenchChem are intended solely for informational purposes. The products available for purchase on BenchChem are specifically designed for in-vitro studies, which are conducted outside of living organisms. In-vitro studies, derived from the Latin term "in glass," involve experiments performed in controlled laboratory settings using cells or tissues. It is important to note that these products are not categorized as medicines or drugs, and they have not received approval from the FDA for the prevention, treatment, or cure of any medical condition, ailment, or disease. We must emphasize that any form of bodily introduction of these products into humans or animals is strictly prohibited by law. It is essential to adhere to these guidelines to ensure compliance with legal and ethical standards in research and experimentation.