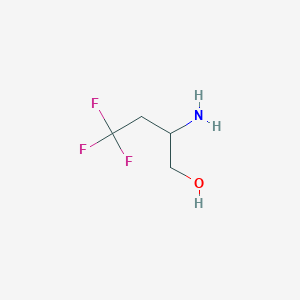
2-amino-4,4,4-trifluoro-1-Butanol
Overview
Description
2-Amino-4,4,4-trifluoro-1-butanol is a fluorinated amino alcohol with the molecular formula C₄H₈F₃NO. Its structure features a hydroxyl (-OH) group at position 1, an amino (-NH₂) group at position 2, and a trifluoromethyl (-CF₃) group at position 4 (Figure 1). This combination of polar (amino, hydroxyl) and lipophilic (trifluoro) groups makes it a versatile candidate for pharmaceutical and agrochemical applications, where fluorine atoms often enhance metabolic stability and bioavailability.
Preparation Methods
Synthetic Routes and Reaction Conditions
The synthesis of 2-amino-4,4,4-trifluoro-1-Butanol can be achieved through several methods. One common approach involves the alkylation of a glycine Schiff base with trifluoromethyl iodide (CF3-CH2-I) under basic conditions. This reaction forms a Ni(II) complex, which is subsequently disassembled to yield the desired product .
Industrial Production Methods
For large-scale production, the method employs a recyclable chiral auxiliary to form the corresponding Ni(II) complex with glycine Schiff base. This complex is alkylated with trifluoromethyl iodide under basic conditions. The resultant alkylated Ni(II) complex is then disassembled to reclaim the chiral auxiliary and produce this compound .
Chemical Reactions Analysis
Oxidation Reactions
The primary amino group undergoes oxidation under controlled conditions:
-
Reagents : Hydrogen peroxide (H₂O₂), potassium permanganate (KMnO₄), or enzymatic systems.
-
Products : Forms imine oxides or nitroxides, which are intermediates for bioactive molecules .
Example Reaction :
Applications: Oxidized derivatives enhance metabolic stability in drug candidates.
Substitution Reactions
The hydroxyl group participates in nucleophilic substitution:
-
Conditions : Catalyzed by acids (H₂SO₄) or bases (KOH).
-
Leaving Groups : –OH replaced by halides (–Cl, –Br) or amines .
Key Pathway :
Applications: Chlorinated analogs serve as intermediates for kinase inhibitors.
Condensation Reactions
The amino and hydroxyl groups facilitate cyclocondensation:
-
Partners : Aldehydes, ketones, or carboxylic acids.
-
Products : Five- or six-membered heterocycles (e.g., oxazolines, pyrimidines) .
Example :
Condensation with benzaldehyde forms a tricyclic oxazoline derivative:
Applications: Antimicrobial agents and enzyme inhibitors.
Esterification and Acylation
The hydroxyl group reacts with acylating agents:
Reaction Table :
Reactant | Conditions | Product | Application |
---|---|---|---|
Acetic anhydride | 80°C, H₂SO₄ | 2-Amino-4,4,4-trifluoro-1-acetate | Prodrug synthesis |
Acryloyl chloride | RT, DMF, base | Acrylate derivative | Polymer crosslinkers |
Peptide Incorporation
The compound acts as a leucine bioisostere in peptide synthesis:
-
Method : Solid-phase peptide synthesis (SPPS) using Fmoc-protected derivatives .
-
Mechanism : Mimics leucine’s steric bulk while enhancing metabolic resistance via CF₃ .
Case Study :
(S)-N-Fmoc-2-amino-4,4,4-trifluorobutanoic acid (synthesized via Ni(II)-complex alkylation) incorporates into peptides with >99% enantiomeric purity .
Reduction and Hydrogenolysis
The trifluoromethyl group stabilizes radicals during reductions:
-
Reagents : Sodium borohydride (NaBH₄), lithium aluminum hydride (LiAlH₄).
Example :
Applications: Surfactants or fluorinated polymers .
Stereochemical Transformations
Enantioselective reactions leverage chiral nickel complexes:
-
Catalyst : Ni(II)-(S)-BPB/(S)-proline complexes.
-
Outcome : Enantiomerically pure (S)- or (R)-isomers for asymmetric drug synthesis .
Optimized Conditions :
Stability and Degradation
-
Hydrolysis : Resistant under neutral pH but degrades in strong acids/bases to form trifluoroacetic acid derivatives .
This compound’s reactivity profile underscores its versatility in synthesizing fluorinated pharmaceuticals, agrochemicals, and materials. Future research may explore its catalytic asymmetric reactions and bioconjugation techniques.
Scientific Research Applications
Chemistry
2-Amino-4,4,4-trifluoro-1-butanol serves as a crucial building block in the synthesis of various fluorinated compounds. Its unique properties allow it to act as a reagent in organic synthesis processes.
Biology
In biological research, this compound is employed to study enzyme mechanisms and protein-ligand interactions. Its fluorinated structure offers insights into how modifications can affect biological activity.
Medicine
The compound is investigated for its potential in drug design as a bioisostere of leucine moiety. This application is particularly relevant in developing pharmaceuticals with enhanced metabolic stability and bioactivity. It has been noted for its ability to mimic natural amino acids while providing improved pharmacological properties .
Case Study 1: Drug Development
Research has demonstrated that derivatives of this compound can be utilized in designing peptides and peptidomimetics that exhibit favorable biological activity. For instance, studies have shown that incorporating this compound into peptide structures can enhance their binding affinity and selectivity towards specific biological targets .
Case Study 2: Specialty Chemicals
In industrial applications, this compound has been used in producing specialty chemicals with enhanced properties. These chemicals are often utilized in materials science for developing advanced materials that require specific thermal or chemical stability .
Comparative Data Table
Application Area | Specific Use | Benefits |
---|---|---|
Chemistry | Building block for fluorinated compounds | Enhances reactivity and stability |
Biology | Study of enzyme mechanisms | Provides insights into protein interactions |
Medicine | Drug design (bioisostere of leucine) | Improved metabolic stability and bioactivity |
Industry | Production of specialty chemicals | Development of advanced materials |
Mechanism of Action
The mechanism of action of 2-amino-4,4,4-trifluoro-1-Butanol involves its interaction with molecular targets through hydrogen bonding and hydrophobic interactions. The trifluoromethyl group enhances the compound’s lipophilicity, allowing it to interact more effectively with hydrophobic pockets in proteins and enzymes. This interaction can modulate the activity of the target proteins, leading to various biological effects .
Comparison with Similar Compounds
Comparison with Structurally Related Compounds
4,4,4-Trifluoro-1-Butanol (TB)
- Structure: Lacks the amino group at position 2 (molecular formula: C₄H₇F₃O).
- Physicochemical Properties: logP: +0.91 (indicating moderate lipophilicity). Polarity: Lower than the amino analog due to the absence of the -NH₂ group.
- Applications: Used as a solvent or intermediate in fluoroorganic synthesis. The absence of the amino group limits its utility in bioactivity-driven contexts compared to 2-amino-4,4,4-trifluoro-1-butanol.
2-Amino-4,4,4-Trifluoro-2-Methylbutan-1-Ol
- Structure: A methyl-branched analog (molecular formula: C₅H₁₀F₃NO).
- No direct data on logP or bioactivity are available, but the amino and trifluoro groups suggest comparable polarity to the target compound.
5-Fluoro-2-Amino-4,6-Dichloropyrimidine
- Structure: An aromatic heterocycle with amino, fluoro, and chloro substituents.
- Biological Activity: Demonstrates potent inhibition of nitric oxide (NO) production (IC₅₀ = 2 μM), attributed to the electron-withdrawing effects of fluorine.
- Relevance: Highlights the role of fluorine in enhancing bioactivity, a property likely shared by this compound.
Comparative Data Table
Key Insights from Functional Group Analysis
- Amino Group (-NH₂): Introduces polarity, reducing logP compared to TB. Enhances hydrogen-bonding capacity, critical for target binding in drug design.
- Trifluoromethyl Group (-CF₃): Increases lipophilicity and metabolic stability. Synergizes with amino groups to balance solubility and membrane permeability.
- Fluorine Substitution: As seen in 5-fluoro-2-amino-4,6-dichloropyrimidine, fluorine enhances bioactivity through electronic and steric effects, a trend expected to extend to this compound.
Biological Activity
2-Amino-4,4,4-trifluoro-1-butanol is a fluorinated alcohol that has garnered attention for its potential biological activities and applications in various fields, including medicinal chemistry and environmental science. This compound features a trifluoromethyl group, which significantly influences its chemical reactivity and interactions with biological systems.
Chemical Structure and Properties
- Chemical Formula : C5H9F3N
- Molecular Weight : 155.13 g/mol
- IUPAC Name : this compound
The presence of fluorine atoms enhances the compound's lipophilicity, allowing it to interact effectively with hydrophobic regions of proteins and enzymes, which may modulate their activity.
The biological activity of this compound is primarily attributed to its ability to form hydrogen bonds and interact with various biomolecules. The trifluoromethyl group enhances the stability and reactivity of the compound, making it a valuable candidate for further research in pharmacology and biochemistry.
Case Studies and Research Findings
- Antimicrobial Activity : Research has indicated that compounds similar to this compound exhibit significant antimicrobial properties against various pathogens. For instance, studies have shown that fluorinated compounds can inhibit the growth of Gram-negative bacteria such as Acinetobacter baumannii and Pseudomonas aeruginosa .
- Toxicological Assessments : A study evaluating the toxicological profile of related compounds revealed that while some derivatives exhibited hepatotoxicity at high doses, others demonstrated favorable safety profiles. The no observed adverse effect level (NOAEL) was determined to be 50 mg/kg body weight per day for certain derivatives .
- Pharmacological Potential : The unique molecular structure of this compound suggests potential applications as a precursor in pharmaceutical synthesis. Its ability to modulate enzyme activity could lead to the development of new therapeutic agents targeting specific diseases .
Comparative Analysis with Similar Compounds
Compound Name | Structure Type | Biological Activity |
---|---|---|
This compound | Fluorinated alcohol | Antimicrobial and potential drug precursor |
2-Methyl-4,4,4-trifluorobutanal | Fluorinated aldehyde | Antimicrobial activity |
2-Amino-4,4,4-trifluorobutyric acid | Amino acid | Neuroprotective effects |
Q & A
Basic Research Questions
Q. What are the optimal synthetic routes for 2-amino-4,4,4-trifluoro-1-butanol, and how can reaction conditions be optimized for yield and purity?
- Methodological Answer : Synthesis typically involves fluorination of precursors like 4,4,4-trifluoro-1-butanol derivatives. For example, nucleophilic substitution or catalytic fluorination using trifluoromethylating agents (e.g., Ruppert-Prakash reagent) can introduce fluorine atoms. Optimization includes temperature control (e.g., -78°C for electrophilic fluorination), solvent selection (e.g., anhydrous THF or DMF), and purification via fractional distillation or column chromatography to isolate the product . Monitoring intermediates via and GC-MS ensures stepwise fidelity.
Q. How can structural elucidation of this compound be achieved using crystallographic and spectroscopic techniques?
- Methodological Answer : Single-crystal X-ray diffraction (SXRD) with SHELX software (e.g., SHELXL for refinement) resolves stereochemistry and bond angles . Complementary techniques include , , and to confirm functional groups and fluorine coupling patterns. High-resolution mass spectrometry (HRMS) validates molecular weight, while IR spectroscopy identifies NH and OH stretches. For labile compounds, low-temperature crystallography (<200 K) minimizes thermal motion artifacts .
Q. What purification strategies are effective for isolating this compound from complex reaction mixtures?
- Methodological Answer : Liquid-liquid extraction (e.g., using dichloromethane/water) removes polar byproducts. Silica gel chromatography with gradients of ethyl acetate/hexane separates enantiomers or diastereomers. For fluorinated compounds, reverse-phase HPLC with C18 columns and trifluoroacetic acid (TFA) as a modifier improves resolution. Recrystallization in ethanol/water mixtures enhances purity, monitored by melting point analysis .
Advanced Research Questions
Q. How do computational methods (DFT, MD simulations) predict the physicochemical properties of this compound, and how do they align with experimental data?
- Methodological Answer : Density Functional Theory (DFT) at the B3LYP/6-311+G(d,p) level calculates dipole moments, Fukui indices for reactivity, and H-bonding propensity. Molecular dynamics (MD) simulations in explicit solvents (e.g., water, DMSO) predict solubility and diffusion coefficients. Discrepancies between computed and experimental shifts may arise from solvent effects or crystal packing, requiring corrections via implicit solvent models (e.g., PCM) .
Q. What strategies resolve contradictions between spectroscopic data (e.g., unexpected splitting) and proposed structures?
- Methodological Answer : Contradictions often stem from dynamic effects (e.g., rotational barriers, tautomerism). Variable-temperature NMR (VT-NMR) between 25°C and -40°C identifies slow exchange processes. Isotopic labeling (e.g., for amino groups) clarifies coupling networks. For fluorine, detects spatial proximity to resolve stereochemical ambiguities .
Q. How can the compound’s stability under varying pH and temperature conditions be systematically evaluated for application in catalytic or biological studies?
- Methodological Answer : Accelerated stability studies involve incubating the compound in buffers (pH 1–13) at 25–60°C. Degradation products are tracked via LC-MS, while Arrhenius plots predict shelf life. For fluorinated alcohols, oxidative stability is assessed using HO or UV light. Thermodynamic parameters (ΔH, ΔS) derived from DSC/TGA quantify phase transitions and decomposition pathways .
Q. What role does the trifluoromethyl group play in modulating the compound’s bioactivity or interaction with enzymes, and how can this be validated experimentally?
- Methodological Answer : Fluorine’s electronegativity alters pKa (e.g., NH group) and lipophilicity (logP). Isothermal titration calorimetry (ITC) quantifies binding affinities with target proteins (e.g., enzymes). Competitive inhibition assays using fluorinated vs. non-fluorinated analogs reveal steric/electronic effects. X-ray co-crystallography identifies fluorine-protein interactions (e.g., C-F⋯H-N hydrogen bonds) .
Properties
IUPAC Name |
2-amino-4,4,4-trifluorobutan-1-ol | |
---|---|---|
Details | Computed by LexiChem 2.6.6 (PubChem release 2019.06.18) | |
Source | PubChem | |
URL | https://pubchem.ncbi.nlm.nih.gov | |
Description | Data deposited in or computed by PubChem | |
InChI |
InChI=1S/C4H8F3NO/c5-4(6,7)1-3(8)2-9/h3,9H,1-2,8H2 | |
Details | Computed by InChI 1.0.5 (PubChem release 2019.06.18) | |
Source | PubChem | |
URL | https://pubchem.ncbi.nlm.nih.gov | |
Description | Data deposited in or computed by PubChem | |
InChI Key |
CYRIDDDXXMMLMU-UHFFFAOYSA-N | |
Details | Computed by InChI 1.0.5 (PubChem release 2019.06.18) | |
Source | PubChem | |
URL | https://pubchem.ncbi.nlm.nih.gov | |
Description | Data deposited in or computed by PubChem | |
Canonical SMILES |
C(C(CO)N)C(F)(F)F | |
Details | Computed by OEChem 2.1.5 (PubChem release 2019.06.18) | |
Source | PubChem | |
URL | https://pubchem.ncbi.nlm.nih.gov | |
Description | Data deposited in or computed by PubChem | |
Molecular Formula |
C4H8F3NO | |
Details | Computed by PubChem 2.1 (PubChem release 2019.06.18) | |
Source | PubChem | |
URL | https://pubchem.ncbi.nlm.nih.gov | |
Description | Data deposited in or computed by PubChem | |
Molecular Weight |
143.11 g/mol | |
Details | Computed by PubChem 2.1 (PubChem release 2021.05.07) | |
Source | PubChem | |
URL | https://pubchem.ncbi.nlm.nih.gov | |
Description | Data deposited in or computed by PubChem | |
Synthesis routes and methods I
Procedure details
Synthesis routes and methods II
Procedure details
Synthesis routes and methods III
Procedure details
Synthesis routes and methods IV
Procedure details
Synthesis routes and methods V
Procedure details
Disclaimer and Information on In-Vitro Research Products
Please be aware that all articles and product information presented on BenchChem are intended solely for informational purposes. The products available for purchase on BenchChem are specifically designed for in-vitro studies, which are conducted outside of living organisms. In-vitro studies, derived from the Latin term "in glass," involve experiments performed in controlled laboratory settings using cells or tissues. It is important to note that these products are not categorized as medicines or drugs, and they have not received approval from the FDA for the prevention, treatment, or cure of any medical condition, ailment, or disease. We must emphasize that any form of bodily introduction of these products into humans or animals is strictly prohibited by law. It is essential to adhere to these guidelines to ensure compliance with legal and ethical standards in research and experimentation.