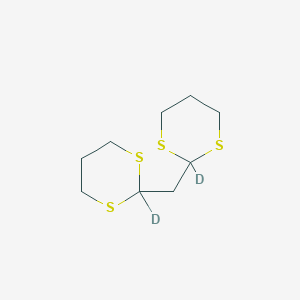
Bis(1,3-dithian-2-yl)methane-d2
Overview
Description
Bis(1,3-dithian-2-yl)methane-d2 (CAS: 105479-87-6) is a deuterated derivative of bis(1,3-dithian-2-yl)methane, where two hydrogen atoms are replaced with deuterium. Its molecular formula is C₉H₁₄D₂S₄, with a molar mass of 254.5 g/mol . The compound is an off-white solid soluble in chloroform, dichloromethane, and ethyl acetate . It is primarily used in research settings, particularly in isotopic labeling studies, to investigate reaction mechanisms or metabolic pathways where deuterium’s kinetic isotope effects are critical .
Preparation Methods
Synthetic Routes and Reaction Conditions: The synthesis of Bis(1,3-dithian-2-yl)methane-d2 typically involves the deuterium exchange of Bis(1,3-dithian-2-yl)methane. This process can be achieved through the reaction of Bis(1,3-dithian-2-yl)methane with deuterium oxide (D2O) under specific conditions. The reaction is usually carried out in the presence of a catalyst to facilitate the exchange of hydrogen atoms with deuterium atoms.
Industrial Production Methods: While the industrial production methods for this compound are not extensively documented, it is likely that the process involves large-scale deuterium exchange reactions similar to those used in laboratory settings. The compound is then purified and characterized to ensure its suitability for research applications.
Chemical Reactions Analysis
Types of Reactions: Bis(1,3-dithian-2-yl)methane-d2 can undergo various chemical reactions, including:
Oxidation: The compound can be oxidized to form sulfoxides or sulfones.
Reduction: Reduction reactions can convert the dithiane rings to thiol groups.
Substitution: The compound can participate in nucleophilic substitution reactions, where the dithiane rings act as leaving groups.
Common Reagents and Conditions:
Oxidation: Common oxidizing agents include hydrogen peroxide (H2O2) and m-chloroperbenzoic acid (m-CPBA).
Reduction: Reducing agents such as lithium aluminum hydride (LiAlH4) or sodium borohydride (NaBH4) are typically used.
Substitution: Nucleophiles like Grignard reagents or organolithium compounds are often employed.
Major Products:
Oxidation: Sulfoxides and sulfones.
Reduction: Thiol groups.
Substitution: Various substituted dithiane derivatives.
Scientific Research Applications
Chemistry: In organic chemistry, Bis(1,3-dithian-2-yl)methane-d2 is used as a building block for the synthesis of more complex molecules. Its deuterium labeling makes it valuable for studying reaction mechanisms and kinetics using nuclear magnetic resonance (NMR) spectroscopy.
Biology and Medicine: The compound is used in metabolic research to trace the pathways of biochemical reactions in vivo. Its stable isotope labeling allows for precise tracking of metabolic processes without altering the biological activity of the molecules involved.
Mechanism of Action
The mechanism of action of Bis(1,3-dithian-2-yl)methane-d2 is primarily related to its role as a labeled compound in research. The deuterium atoms in the molecule allow for the tracking of the compound through various chemical and biological processes. This enables researchers to study the pathways and mechanisms of reactions with high precision.
Comparison with Similar Compounds
Comparison with Structurally Similar Compounds
Bis(1,3-dithian-2-yl)methane (Non-Deuterated)
- CAS: Not explicitly provided, but referenced as the parent compound.
- Molecular Formula : C₉H₁₆S₄ (vs. C₉H₁₄D₂S₄ for the deuterated form).
- Key Differences: Isotopic Substitution: The non-deuterated form lacks deuterium, resulting in a lower molecular weight (248.5 g/mol vs. 254.5 g/mol) . Reactivity: Deuterium substitution may reduce reaction rates in processes involving C–H(D) bond cleavage due to kinetic isotope effects. For example, in acid-catalyzed reactions, the deuterated compound might exhibit slower proton (deuteron) transfer . Spectroscopic Properties: The deuterated form shows distinct NMR signals (e.g., absence of proton peaks at deuterated sites) and altered IR vibrational modes .
Bis(1,3-dithian-2-yl)methane-d (Monodeuterated)
- CAS : 31401-53-3
- Molecular Formula : C₉H₁₅DS₄ (vs. C₉H₁₄D₂S₄ for the d2 form).
- Key Differences: Deuterium Count: The monodeuterated variant contains one deuterium atom, leading to intermediate molecular weight (250.5 g/mol) . Applications: Used in studies requiring partial isotopic labeling, whereas the d2 form provides higher isotopic enrichment for precise mechanistic tracking .
(S)-1-(1,3-Dithian-2-yl)-2-propanol
- Relevance : A structurally related dithiane derivative used as a chiral intermediate in synthesizing (S,S)-grahamimycin A1 .
- Key Differences :
- Functional Groups : Contains a hydroxyl group, enabling participation in esterification or oxidation reactions, unlike the inert methane bridge in Bis(1,3-dithian-2-yl)methane-d2.
- Stereochemical Utility : The dithiane moiety stabilizes carbocation intermediates in asymmetric synthesis, a property less relevant to the deuterated methane derivative .
Comparative Data Table
Compound | CAS | Molecular Formula | Molar Mass (g/mol) | Key Applications |
---|---|---|---|---|
This compound | 105479-87-6 | C₉H₁₄D₂S₄ | 254.5 | Isotopic labeling, mechanistic studies |
Bis(1,3-dithian-2-yl)methane | N/A | C₉H₁₆S₄ | 248.5 | General organic synthesis |
Bis(1,3-dithian-2-yl)methane-d | 31401-53-3 | C₉H₁₅DS₄ | 250.5 | Partial isotopic labeling |
(S)-1-(1,3-dithian-2-yl)-2-propanol | N/A | C₇H₁₂OS₂ | 176.3 | Chiral synthesis of antibiotics |
Biological Activity
Bis(1,3-dithian-2-yl)methane-d2 is a deuterated compound that has garnered attention in biochemical research due to its unique properties and applications in metabolic studies. This article explores the biological activity of this compound, focusing on its mechanisms, applications in research, and comparative analysis with similar compounds.
- Molecular Formula : C9H14D2S4
- Molecular Weight : 254.50 g/mol
- CAS Number : 14947-51-4
The compound is characterized by its stable isotope labeling with deuterium, which allows for enhanced tracking of biochemical processes without altering the biological activity of the molecules involved.
The primary mechanism of action for this compound is its role as a labeled compound in metabolic research. The deuterium atoms facilitate the tracking of this molecule through various chemical and biological processes, enabling researchers to study reaction pathways and mechanisms with high precision.
Key Reactions:
- Oxidation : Forms sulfoxides and sulfones.
- Reduction : Produces thiol groups.
- Substitution : Generates various substituted dithiane derivatives.
Applications in Biological Research
This compound is primarily utilized in the following areas:
- Metabolic Research : The compound is instrumental in tracing metabolic pathways in vivo. Its stable isotope labeling allows for precise tracking of metabolic processes, making it valuable for understanding biochemical reactions without interference.
- Nuclear Magnetic Resonance (NMR) Spectroscopy : It serves as an internal standard due to its high symmetry and lack of interfering peaks, facilitating accurate chemical shift calibration and signal intensity normalization.
- Protein Interaction Studies : The compound can modify thiol groups within proteins, enhancing their stability and detectability during various biological assays. This property aids in studying protein-protein interactions using NMR-based paramagnetic relaxation enhancement (PRE).
Comparative Analysis
A comparison with similar compounds highlights the unique features of this compound:
Compound | Deuterated | Applications | Unique Features |
---|---|---|---|
Bis(1,3-dithian-2-yl)methane | No | Metabolic studies | Non-deuterated version; lacks tracking capabilities |
1,3-Dithiane | No | Organic synthesis | Simpler structure; lacks methylene bridge |
2,2'-Methylenebis(1,3-dithiane) | No | Synthesis of complex molecules | Different substitution pattern |
The primary advantage of this compound lies in its deuterium labeling, which enhances its utility in research applications requiring precise molecular tracking.
Case Studies
Several studies have utilized this compound to explore its biological activity:
- Study on Metabolic Pathways : Researchers employed this compound to trace the metabolic pathways of specific substrates in living organisms. The results indicated that the compound effectively tracked the transformation of substrates into products without altering their natural metabolic rates.
- Protein Interaction Dynamics : A study investigated the dynamics of protein interactions using NMR techniques. By incorporating this compound into specific sites on proteins, researchers could observe changes in relaxation rates that provided insights into interaction mechanisms.
Q & A
Q. What are the optimal synthetic routes for Bis(1,3-dithian-2-yl)methane-d2, and how can purity be ensured?
Basic Research Question
The synthesis of deuterated compounds like this compound requires careful selection of precursors and reaction conditions. A pre-experimental design (e.g., pre-test/post-test with control groups) can identify critical variables such as solvent polarity, temperature, and deuteration efficiency . Factorial design methodologies allow systematic variation of these parameters to optimize yield and isotopic incorporation . Purity validation typically involves high-resolution mass spectrometry (HRMS) and deuterium NMR to confirm isotopic enrichment and absence of protonated byproducts. Cross-referencing with literature on analogous dithiane ligands ensures alignment with established synthetic protocols .
Q. How does deuteration impact the compound’s reactivity in coordination chemistry?
Advanced Research Question
Deuterium substitution alters vibrational frequencies and electron density distribution, potentially affecting metal-ligand bond strength and reaction kinetics. To investigate this, researchers should:
- Compare kinetic isotope effects (KIEs) in ligand substitution reactions using protonated vs. deuterated analogs.
- Employ ultrafast spectroscopy (e.g., time-resolved IR) to track transient intermediates .
- Integrate computational models (e.g., DFT calculations) to correlate isotopic effects with electronic structure changes .
Contradictions in observed KIEs may arise from competing reaction pathways, necessitating mechanistic studies via Eyring analysis .
Q. What spectroscopic techniques are most effective for characterizing this compound?
Basic Research Question
Deuterium-enriched compounds require specialized techniques:
- ²H NMR : Resolves deuterium environments, with chemical shifts sensitive to sulfur coordination geometry .
- Raman Spectroscopy : Highlights vibrational modes of the dithiane rings, with deuteration-induced frequency shifts (~10–15 cm⁻¹) confirming isotopic substitution .
- X-ray Crystallography : Resolves structural distortions caused by deuterium’s smaller atomic radius compared to hydrogen. Ensure low-temperature data collection to mitigate thermal motion artifacts .
Q. How can computational models predict isotopic effects in this compound-mediated reactions?
Advanced Research Question
Combining molecular dynamics (MD) and quantum mechanics/molecular mechanics (QM/MM) simulations enables prediction of deuteration effects on reaction pathways. Key steps:
Parameterize force fields using experimental vibrational spectra.
Simulate isotopic substitution’s impact on transition-state geometries.
Validate models against experimental KIEs and isotopic scrambling data .
Discrepancies between simulation and experimental data may indicate overlooked solvent effects or non-adiabatic transitions, requiring iterative refinement .
Q. How should researchers design experiments to study this compound’s role as a sulfur-donor ligand?
Basic Research Question
A mixed-methods approach is recommended:
- Factorial Design : Vary metal centers (e.g., Pd, Pt) and solvent systems to assess ligand binding affinity .
- Spectroscopic Titrations : Use UV-vis or NMR to determine stability constants (logβ) for metal complexes .
- Theoretical Framework : Link results to Hard-Soft Acid-Base (HSAB) theory to explain selectivity trends .
Contradictory logβ values across studies may stem from solvent polarity or counterion effects, necessitating controlled replication .
Q. What strategies resolve contradictions in kinetic data from isotopic substitution studies?
Advanced Research Question
Conflicting kinetic data often arise from:
- Isotopic Purity Issues : Ensure deuterated samples are >98% enriched via HRMS validation .
- Hidden Variables : Use sensitivity analysis to identify overlooked factors (e.g., trace moisture in reactions) .
- Multi-probe Experiments : Combine kinetic assays with in-situ FTIR or EPR to detect intermediate species .
Theoretical reconciliation involves revisiting the reaction’s conceptual framework (e.g., Marcus theory for electron transfer) to align divergent data .
Q. How can researchers leverage AI to optimize reaction conditions for deuterated ligand synthesis?
Advanced Research Question
AI-driven platforms like COMSOL Multiphysics integrate reaction kinetics datasets to predict optimal deuteration conditions:
- Train models on historical data for dithiane ligand synthesis.
- Incorporate real-time feedback from inline analytics (e.g., Raman probes) to adjust parameters dynamically .
- Validate predictions via high-throughput robotic screening, minimizing human bias .
Challenges include dataset scarcity for niche deuterated compounds, necessitating collaborative data-sharing initiatives .
Properties
IUPAC Name |
2-deuterio-2-[(2-deuterio-1,3-dithian-2-yl)methyl]-1,3-dithiane | |
---|---|---|
Source | PubChem | |
URL | https://pubchem.ncbi.nlm.nih.gov | |
Description | Data deposited in or computed by PubChem | |
InChI |
InChI=1S/C9H16S4/c1-3-10-8(11-4-1)7-9-12-5-2-6-13-9/h8-9H,1-7H2/i8D,9D | |
Source | PubChem | |
URL | https://pubchem.ncbi.nlm.nih.gov | |
Description | Data deposited in or computed by PubChem | |
InChI Key |
DCJKPLNHQJEQOL-XETGXLELSA-N | |
Source | PubChem | |
URL | https://pubchem.ncbi.nlm.nih.gov | |
Description | Data deposited in or computed by PubChem | |
Canonical SMILES |
C1CSC(SC1)CC2SCCCS2 | |
Source | PubChem | |
URL | https://pubchem.ncbi.nlm.nih.gov | |
Description | Data deposited in or computed by PubChem | |
Isomeric SMILES |
[2H]C1(SCCCS1)CC2(SCCCS2)[2H] | |
Source | PubChem | |
URL | https://pubchem.ncbi.nlm.nih.gov | |
Description | Data deposited in or computed by PubChem | |
Molecular Formula |
C9H16S4 | |
Source | PubChem | |
URL | https://pubchem.ncbi.nlm.nih.gov | |
Description | Data deposited in or computed by PubChem | |
DSSTOX Substance ID |
DTXSID10483966 | |
Record name | Bis(1,3-dithian-2-yl)methane-d2 | |
Source | EPA DSSTox | |
URL | https://comptox.epa.gov/dashboard/DTXSID10483966 | |
Description | DSSTox provides a high quality public chemistry resource for supporting improved predictive toxicology. | |
Molecular Weight |
254.5 g/mol | |
Source | PubChem | |
URL | https://pubchem.ncbi.nlm.nih.gov | |
Description | Data deposited in or computed by PubChem | |
CAS No. |
105479-87-6 | |
Record name | 1,3-Dithiane-2-d, 2,2′-methylenebis- | |
Source | CAS Common Chemistry | |
URL | https://commonchemistry.cas.org/detail?cas_rn=105479-87-6 | |
Description | CAS Common Chemistry is an open community resource for accessing chemical information. Nearly 500,000 chemical substances from CAS REGISTRY cover areas of community interest, including common and frequently regulated chemicals, and those relevant to high school and undergraduate chemistry classes. This chemical information, curated by our expert scientists, is provided in alignment with our mission as a division of the American Chemical Society. | |
Explanation | The data from CAS Common Chemistry is provided under a CC-BY-NC 4.0 license, unless otherwise stated. | |
Record name | Bis(1,3-dithian-2-yl)methane-d2 | |
Source | EPA DSSTox | |
URL | https://comptox.epa.gov/dashboard/DTXSID10483966 | |
Description | DSSTox provides a high quality public chemistry resource for supporting improved predictive toxicology. | |
Retrosynthesis Analysis
AI-Powered Synthesis Planning: Our tool employs the Template_relevance Pistachio, Template_relevance Bkms_metabolic, Template_relevance Pistachio_ringbreaker, Template_relevance Reaxys, Template_relevance Reaxys_biocatalysis model, leveraging a vast database of chemical reactions to predict feasible synthetic routes.
One-Step Synthesis Focus: Specifically designed for one-step synthesis, it provides concise and direct routes for your target compounds, streamlining the synthesis process.
Accurate Predictions: Utilizing the extensive PISTACHIO, BKMS_METABOLIC, PISTACHIO_RINGBREAKER, REAXYS, REAXYS_BIOCATALYSIS database, our tool offers high-accuracy predictions, reflecting the latest in chemical research and data.
Strategy Settings
Precursor scoring | Relevance Heuristic |
---|---|
Min. plausibility | 0.01 |
Model | Template_relevance |
Template Set | Pistachio/Bkms_metabolic/Pistachio_ringbreaker/Reaxys/Reaxys_biocatalysis |
Top-N result to add to graph | 6 |
Feasible Synthetic Routes
Disclaimer and Information on In-Vitro Research Products
Please be aware that all articles and product information presented on BenchChem are intended solely for informational purposes. The products available for purchase on BenchChem are specifically designed for in-vitro studies, which are conducted outside of living organisms. In-vitro studies, derived from the Latin term "in glass," involve experiments performed in controlled laboratory settings using cells or tissues. It is important to note that these products are not categorized as medicines or drugs, and they have not received approval from the FDA for the prevention, treatment, or cure of any medical condition, ailment, or disease. We must emphasize that any form of bodily introduction of these products into humans or animals is strictly prohibited by law. It is essential to adhere to these guidelines to ensure compliance with legal and ethical standards in research and experimentation.