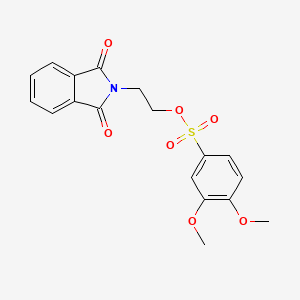
2-(1,3-Dioxoisoindolin-2-yl)ethyl 3,4-dimethoxybenzenesulfonate
Overview
Description
2-(1,3-Dioxoisoindolin-2-yl)ethyl 3,4-dimethoxybenzenesulfonate is an organic compound characterized by the presence of an isoindoline-1,3-dione moiety and a benzenesulfonate group
Mechanism of Action
Target of Action
The primary targets of 2-(1,3-Dioxoisoindolin-2-yl)ethyl 3,4-dimethoxybenzenesulfonate It is known that compounds with an isoindoline nucleus and carbonyl groups at positions 1 and 3, such as this compound, have gained significant attention for their potential use in diverse fields . They have shown potential as therapeutic agents .
Mode of Action
The exact mode of action of This compound It is likely that the compound interacts with its targets through the isoindoline nucleus and carbonyl groups at positions 1 and 3.
Biochemical Pathways
The specific biochemical pathways affected by This compound It is known that n-isoindoline-1,3-dione heterocycles have diverse chemical reactivity and promising applications .
Pharmacokinetics
The ADME properties (Absorption, Distribution, Metabolism, and Excretion) of This compound It is known that the compound is a solid at room temperature and can dissolve in some organic solvents, which may influence its bioavailability.
Result of Action
The specific molecular and cellular effects of This compound It is known that n-isoindoline-1,3-dione derivatives have shown potential as therapeutic agents .
Action Environment
The influence of environmental factors on the action, efficacy, and stability of This compound It is known that the compound is a solid at room temperature and can dissolve in some organic solvents, which may influence its action in different environments.
Biochemical Analysis
Biochemical Properties
2-(1,3-Dioxoisoindolin-2-yl)ethyl 3,4-dimethoxybenzenesulfonate plays a significant role in biochemical reactions, particularly in the context of enzyme interactions and protein modifications. This compound is known to interact with enzymes such as human cytosolic thymidine kinase (hTK-1), which is involved in the pyrimidine salvage pathway . The interaction with hTK-1 leads to the phosphorylation of nucleosides to their corresponding 5’-monophosphates, thereby influencing nucleotide metabolism. Additionally, the compound’s sulfonate group allows it to form stable complexes with various proteins, potentially altering their function and activity.
Cellular Effects
The effects of this compound on cellular processes are multifaceted. This compound has been shown to influence cell signaling pathways, gene expression, and cellular metabolism. For instance, its interaction with hTK-1 can lead to increased nucleotide synthesis in proliferating cells, thereby promoting cell growth and division . Furthermore, the compound’s ability to form complexes with proteins can modulate signaling pathways, potentially affecting processes such as apoptosis and cell differentiation.
Molecular Mechanism
At the molecular level, this compound exerts its effects through several mechanisms. The compound’s phthalimide group allows it to bind to specific enzyme active sites, leading to enzyme inhibition or activation . For example, its interaction with hTK-1 results in the phosphorylation of nucleosides, which is a crucial step in nucleotide metabolism. Additionally, the compound’s sulfonate group can form electrostatic interactions with positively charged amino acid residues in proteins, thereby influencing protein structure and function.
Temporal Effects in Laboratory Settings
In laboratory settings, the effects of this compound have been observed to change over time. The compound is relatively stable under standard laboratory conditions, but it can undergo degradation when exposed to extreme pH or temperature . Long-term studies have shown that the compound can have sustained effects on cellular function, particularly in terms of nucleotide metabolism and protein interactions. The extent of these effects can vary depending on the specific experimental conditions.
Dosage Effects in Animal Models
The effects of this compound in animal models are dose-dependent. At lower doses, the compound has been shown to promote cell proliferation and nucleotide synthesis without causing significant toxicity . At higher doses, it can lead to adverse effects such as cytotoxicity and disruption of normal cellular processes. These threshold effects highlight the importance of careful dosage optimization in experimental studies.
Metabolic Pathways
This compound is involved in several metabolic pathways, primarily those related to nucleotide metabolism. The compound’s interaction with hTK-1 facilitates the phosphorylation of nucleosides, which is a key step in the pyrimidine salvage pathway . Additionally, the compound can influence metabolic flux by altering the activity of enzymes involved in nucleotide synthesis and degradation.
Transport and Distribution
Within cells and tissues, this compound is transported and distributed through various mechanisms. The compound can interact with transporters and binding proteins, which facilitate its uptake and localization within specific cellular compartments . Its sulfonate group also allows it to accumulate in regions with high protein concentrations, potentially enhancing its biochemical effects.
Subcellular Localization
The subcellular localization of this compound is influenced by its structural properties and interactions with cellular components. The compound is known to localize in the cytoplasm and nucleus, where it can interact with enzymes and proteins involved in nucleotide metabolism and gene expression . Additionally, post-translational modifications such as phosphorylation can further influence its localization and activity within cells.
Preparation Methods
Synthetic Routes and Reaction Conditions
The synthesis of 2-(1,3-Dioxoisoindolin-2-yl)ethyl 3,4-dimethoxybenzenesulfonate typically involves the reaction of 2-(1,3-dioxoisoindolin-2-yl)ethanol with 3,4-dimethoxybenzenesulfonyl chloride. The reaction is carried out in the presence of a base, such as triethylamine, in an organic solvent like dichloromethane. The reaction mixture is stirred at room temperature for several hours, followed by purification through column chromatography to obtain the desired product .
Industrial Production Methods
Industrial production of this compound may involve similar synthetic routes but on a larger scale. The use of continuous flow reactors and automated systems can enhance the efficiency and yield of the production process. Additionally, optimization of reaction conditions, such as temperature, solvent, and catalyst, can further improve the scalability of the synthesis .
Chemical Reactions Analysis
Types of Reactions
2-(1,3-Dioxoisoindolin-2-yl)ethyl 3,4-dimethoxybenzenesulfonate can undergo various chemical reactions, including:
Oxidation: The compound can be oxidized to introduce additional functional groups or modify existing ones.
Reduction: Reduction reactions can be used to convert the isoindoline-1,3-dione moiety to other derivatives.
Substitution: Nucleophilic substitution reactions can occur at the benzenesulfonate group, leading to the formation of new compounds.
Common Reagents and Conditions
Oxidation: Common oxidizing agents include potassium permanganate (KMnO₄) and chromium trioxide (CrO₃).
Reduction: Reducing agents such as lithium aluminum hydride (LiAlH₄) and sodium borohydride (NaBH₄) are often used.
Substitution: Nucleophiles like amines, thiols, and alcohols can react with the benzenesulfonate group under basic conditions.
Major Products Formed
The major products formed from these reactions depend on the specific reagents and conditions used. For example, oxidation may yield sulfonic acids, while reduction can produce isoindoline derivatives. Substitution reactions can result in a variety of functionalized compounds .
Scientific Research Applications
2-(1,3-Dioxoisoindolin-2-yl)ethyl 3,4-dimethoxybenzenesulfonate has several scientific research applications:
Chemistry: It is used as a building block in organic synthesis for the preparation of complex molecules.
Biology: The compound is studied for its potential biological activities, including antimicrobial and anticancer properties.
Medicine: Research is ongoing to explore its use as a pharmaceutical intermediate and its potential therapeutic effects.
Industry: It is utilized in the development of advanced materials, such as polymers and photochromic materials.
Comparison with Similar Compounds
Similar Compounds
- 2-(1,3-Dioxoisoindolin-2-yl)ethyl benzenesulfonate
- 2-(1,3-Dioxoisoindolin-2-yl)ethyl 4-methylbenzenesulfonate
- 2-(1,3-Dioxoisoindolin-2-yl)ethyl 2,4-dimethoxybenzenesulfonate
Uniqueness
2-(1,3-Dioxoisoindolin-2-yl)ethyl 3,4-dimethoxybenzenesulfonate is unique due to the presence of both the isoindoline-1,3-dione and the 3,4-dimethoxybenzenesulfonate groups. This combination imparts distinct chemical and biological properties, making it a valuable compound for various applications. The 3,4-dimethoxy substitution pattern on the benzenesulfonate group can influence the compound’s reactivity and interactions with biological targets, distinguishing it from other similar compounds .
Biological Activity
2-(1,3-Dioxoisoindolin-2-yl)ethyl 3,4-dimethoxybenzenesulfonate is a compound of interest in medicinal chemistry due to its potential biological activities. This article reviews the synthesis, biological activity, structure-activity relationships (SAR), and relevant case studies associated with this compound.
Chemical Structure and Properties
The chemical formula for this compound is C18H17NO7S. It features a dioxoisoindoline core connected to an ethyl group and a sulfonate moiety derived from 3,4-dimethoxybenzene. This unique structure suggests potential interactions with various biological targets.
Synthesis
The synthesis of this compound typically involves multi-step organic reactions that include the formation of the dioxoisoindoline framework followed by sulfonation and etherification processes. The yield and purity of the synthesized compound are critical for subsequent biological evaluations.
Antimicrobial Activity
Recent studies have evaluated the antimicrobial properties of related compounds in the dioxoisoindoline family. For instance, derivatives have shown significant antibacterial activity against Gram-positive bacteria such as Staphylococcus aureus and Enterococcus faecalis .
Compound | Target Bacteria | Minimum Inhibitory Concentration (MIC) |
---|---|---|
Compound A | S. aureus | 625 µg/mL |
Compound B | E. faecalis | 1250 µg/mL |
These findings suggest that modifications in the structure can enhance or diminish antibacterial efficacy.
Receptor Binding Affinity
The binding affinity of similar compounds at dopamine receptors has been investigated using radioligand binding assays. For example, compounds with specific substituents demonstrated varied affinities for D2 and D3 receptors .
Compound | D2 Receptor Affinity (K_i) | D3 Receptor Affinity (K_i) |
---|---|---|
Compound X | 89.2 nM | 21.8 nM |
Compound Y | >1000 nM | >1000 nM |
These results indicate that structural variations significantly impact receptor selectivity and potency.
Study 1: Structure-Activity Relationship Analysis
A comprehensive SAR study focused on the effects of various substituents on the biological activity of dioxoisoindoline derivatives. The study concluded that compounds with electron-donating groups exhibited enhanced binding affinities at dopamine receptors compared to those with electron-withdrawing groups .
Study 2: Antifungal Screening
Another study examined the antifungal properties of related compounds against Candida albicans. The results indicated that specific structural features contributed to increased antifungal activity, with some derivatives showing complete inhibition at lower concentrations .
Properties
IUPAC Name |
2-(1,3-dioxoisoindol-2-yl)ethyl 3,4-dimethoxybenzenesulfonate | |
---|---|---|
Details | Computed by Lexichem TK 2.7.0 (PubChem release 2021.05.07) | |
Source | PubChem | |
URL | https://pubchem.ncbi.nlm.nih.gov | |
Description | Data deposited in or computed by PubChem | |
InChI |
InChI=1S/C18H17NO7S/c1-24-15-8-7-12(11-16(15)25-2)27(22,23)26-10-9-19-17(20)13-5-3-4-6-14(13)18(19)21/h3-8,11H,9-10H2,1-2H3 | |
Details | Computed by InChI 1.0.6 (PubChem release 2021.05.07) | |
Source | PubChem | |
URL | https://pubchem.ncbi.nlm.nih.gov | |
Description | Data deposited in or computed by PubChem | |
InChI Key |
LCZGWILEEZTEJK-UHFFFAOYSA-N | |
Details | Computed by InChI 1.0.6 (PubChem release 2021.05.07) | |
Source | PubChem | |
URL | https://pubchem.ncbi.nlm.nih.gov | |
Description | Data deposited in or computed by PubChem | |
Canonical SMILES |
COC1=C(C=C(C=C1)S(=O)(=O)OCCN2C(=O)C3=CC=CC=C3C2=O)OC | |
Details | Computed by OEChem 2.3.0 (PubChem release 2021.05.07) | |
Source | PubChem | |
URL | https://pubchem.ncbi.nlm.nih.gov | |
Description | Data deposited in or computed by PubChem | |
Molecular Formula |
C18H17NO7S | |
Details | Computed by PubChem 2.1 (PubChem release 2021.05.07) | |
Source | PubChem | |
URL | https://pubchem.ncbi.nlm.nih.gov | |
Description | Data deposited in or computed by PubChem | |
Molecular Weight |
391.4 g/mol | |
Details | Computed by PubChem 2.1 (PubChem release 2021.05.07) | |
Source | PubChem | |
URL | https://pubchem.ncbi.nlm.nih.gov | |
Description | Data deposited in or computed by PubChem | |
Disclaimer and Information on In-Vitro Research Products
Please be aware that all articles and product information presented on BenchChem are intended solely for informational purposes. The products available for purchase on BenchChem are specifically designed for in-vitro studies, which are conducted outside of living organisms. In-vitro studies, derived from the Latin term "in glass," involve experiments performed in controlled laboratory settings using cells or tissues. It is important to note that these products are not categorized as medicines or drugs, and they have not received approval from the FDA for the prevention, treatment, or cure of any medical condition, ailment, or disease. We must emphasize that any form of bodily introduction of these products into humans or animals is strictly prohibited by law. It is essential to adhere to these guidelines to ensure compliance with legal and ethical standards in research and experimentation.