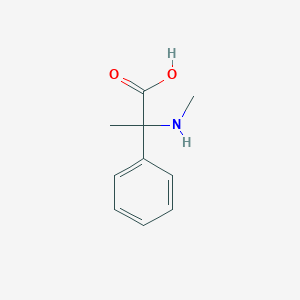
N-methyl-2-phenylalanine
- Click on QUICK INQUIRY to receive a quote from our team of experts.
- With the quality product at a COMPETITIVE price, you can focus more on your research.
Overview
Description
N-methyl-2-phenylalanine: is a derivative of the amino acid phenylalanine, where a methyl group is attached to the nitrogen atom of the amino group. This modification can significantly alter the properties and reactivity of the compound, making it a subject of interest in various fields of scientific research and industrial applications.
Mechanism of Action
Target of Action
N-methyl-2-phenylalanine is a derivative of phenylalanine, an essential aromatic amino acid Phenylalanine is known to be a precursor of melanin, dopamine, noradrenalin (norepinephrine), and thyroxine . Therefore, it can be inferred that this compound might interact with similar targets.
Mode of Action
Phenylalanine, from which this compound is derived, is used by the brain to produce norepinephrine, a chemical that transmits signals between nerve cells and the brain . This suggests that this compound might interact with its targets in a similar manner, leading to changes in neurotransmitter levels and signal transmission.
Biochemical Pathways
This compound likely participates in similar biochemical pathways as phenylalanine. Phenylalanine is metabolized to produce maleylacetoacetate in a four-step biochemical reaction . This process involves the conversion of phenylalanine to tyrosine, which is then used in the production of various compounds, including melanin, dopamine, noradrenalin (norepinephrine), and thyroxine .
Result of Action
Given its similarity to phenylalanine, it might influence neurotransmitter levels and signal transmission in the brain .
Biochemical Analysis
Biochemical Properties
N-methyl-2-phenylalanine participates in several biochemical reactions. It interacts with various enzymes, proteins, and other biomolecules. For instance, phenylalanine is converted into tyrosine by the enzyme phenylalanine hydroxylase . The conversion of phenylalanine to tyrosine is a critical step in the synthesis of adrenaline . This compound, being a derivative of phenylalanine, may also interact with these enzymes and participate in similar biochemical reactions .
Cellular Effects
This compound can influence various types of cells and cellular processes. It may impact cell signaling pathways, gene expression, and cellular metabolism. For example, phenylalanine and its derivatives have been associated with promoting mental alertness and memory, elevating mood, and suppressing appetite . Therefore, this compound might have similar effects on cellular function.
Molecular Mechanism
The molecular mechanism of this compound involves its interactions with biomolecules, enzyme inhibition or activation, and changes in gene expression. For instance, phenylalanine is converted into tyrosine by phenylalanine hydroxylase, which is a mixed-function oxygenase . This compound, as a derivative of phenylalanine, might interact with this enzyme and other biomolecules in a similar manner.
Metabolic Pathways
This compound is likely involved in the same metabolic pathways as phenylalanine. Phenylalanine is converted into tyrosine, which is a precursor for the synthesis of adrenaline . Therefore, this compound might interact with the same enzymes or cofactors and affect metabolic flux or metabolite levels.
Preparation Methods
Synthetic Routes and Reaction Conditions
The synthesis of N-methyl-2-phenylalanine can be achieved through several methods. One common approach involves the reductive methylamination of phenylpyruvate using engineered microorganisms such as Corynebacterium glutamicum . This method involves the use of specific enzymes that facilitate the methylation process, resulting in the production of this compound with high enantiopurity and yield.
Another method involves the N-methylation of phenylalanine using chemical reagents. This can be done through the reaction of phenylalanine with formaldehyde and a reducing agent such as sodium cyanoborohydride . The reaction typically takes place in an aqueous or organic solvent under controlled temperature and pH conditions to ensure the selective methylation of the amino group.
Industrial Production Methods
For industrial-scale production, the fermentative route using engineered microorganisms is often preferred due to its sustainability and efficiency. The process involves the cultivation of genetically modified bacteria in a bioreactor, where they convert phenylpyruvate to this compound in the presence of a methyl donor such as monomethylamine . This method offers advantages such as high yield, low environmental impact, and the ability to produce the compound with high enantiopurity.
Chemical Reactions Analysis
Types of Reactions
N-methyl-2-phenylalanine can undergo various chemical reactions, including:
Oxidation: The compound can be oxidized to form corresponding ketones or aldehydes, depending on the reaction conditions and oxidizing agents used.
Reduction: Reduction reactions can convert this compound to its corresponding amine or alcohol derivatives.
Substitution: The compound can participate in nucleophilic substitution reactions, where the methyl group or the phenyl ring can be substituted with other functional groups.
Common Reagents and Conditions
Oxidation: Common oxidizing agents include potassium permanganate (KMnO4) and chromium trioxide (CrO3) in acidic or basic conditions.
Reduction: Reducing agents such as lithium aluminum hydride (LiAlH4) or sodium borohydride (NaBH4) are commonly used.
Substitution: Reagents such as alkyl halides or acyl chlorides can be used in the presence of a base to facilitate substitution reactions.
Major Products Formed
Oxidation: Formation of N-methyl-2-phenylacetaldehyde or N-methyl-2-phenylacetone.
Reduction: Formation of N-methyl-2-phenylethylamine or N-methyl-2-phenylethanol.
Substitution: Formation of various substituted derivatives depending on the reagents used.
Scientific Research Applications
N-methyl-2-phenylalanine has a wide range of applications in scientific research:
Comparison with Similar Compounds
Similar Compounds
Phenylalanine: The parent compound, which lacks the N-methyl group.
N-methylphenylalanine: A similar compound with a methyl group attached to the nitrogen atom.
2-iodo-L-phenylalanine: A phenylalanine analog with an iodine substituent at the benzene ring.
Uniqueness
N-methyl-2-phenylalanine is unique due to its specific structural modification, which imparts distinct chemical and biological properties. The N-methyl group enhances its lipophilicity, improving its membrane permeability and bioavailability . This makes it a valuable compound for drug delivery applications, particularly in crossing the blood-brain barrier .
Properties
IUPAC Name |
2-(methylamino)-2-phenylpropanoic acid |
Source
|
---|---|---|
Details | Computed by LexiChem 2.6.6 (PubChem release 2019.06.18) | |
Source | PubChem | |
URL | https://pubchem.ncbi.nlm.nih.gov | |
Description | Data deposited in or computed by PubChem | |
InChI |
InChI=1S/C10H13NO2/c1-10(11-2,9(12)13)8-6-4-3-5-7-8/h3-7,11H,1-2H3,(H,12,13) |
Source
|
Details | Computed by InChI 1.0.5 (PubChem release 2019.06.18) | |
Source | PubChem | |
URL | https://pubchem.ncbi.nlm.nih.gov | |
Description | Data deposited in or computed by PubChem | |
InChI Key |
CNCXSFDPQFYHDR-UHFFFAOYSA-N |
Source
|
Details | Computed by InChI 1.0.5 (PubChem release 2019.06.18) | |
Source | PubChem | |
URL | https://pubchem.ncbi.nlm.nih.gov | |
Description | Data deposited in or computed by PubChem | |
Canonical SMILES |
CC(C1=CC=CC=C1)(C(=O)O)NC |
Source
|
Details | Computed by OEChem 2.1.5 (PubChem release 2019.06.18) | |
Source | PubChem | |
URL | https://pubchem.ncbi.nlm.nih.gov | |
Description | Data deposited in or computed by PubChem | |
Molecular Formula |
C10H13NO2 |
Source
|
Details | Computed by PubChem 2.1 (PubChem release 2019.06.18) | |
Source | PubChem | |
URL | https://pubchem.ncbi.nlm.nih.gov | |
Description | Data deposited in or computed by PubChem | |
Molecular Weight |
179.22 g/mol |
Source
|
Details | Computed by PubChem 2.1 (PubChem release 2021.05.07) | |
Source | PubChem | |
URL | https://pubchem.ncbi.nlm.nih.gov | |
Description | Data deposited in or computed by PubChem | |
Disclaimer and Information on In-Vitro Research Products
Please be aware that all articles and product information presented on BenchChem are intended solely for informational purposes. The products available for purchase on BenchChem are specifically designed for in-vitro studies, which are conducted outside of living organisms. In-vitro studies, derived from the Latin term "in glass," involve experiments performed in controlled laboratory settings using cells or tissues. It is important to note that these products are not categorized as medicines or drugs, and they have not received approval from the FDA for the prevention, treatment, or cure of any medical condition, ailment, or disease. We must emphasize that any form of bodily introduction of these products into humans or animals is strictly prohibited by law. It is essential to adhere to these guidelines to ensure compliance with legal and ethical standards in research and experimentation.