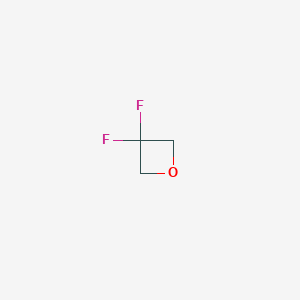
3,3-Difluorooxetane
- Click on QUICK INQUIRY to receive a quote from our team of experts.
- With the quality product at a COMPETITIVE price, you can focus more on your research.
Overview
Description
3,3-Difluorooxetane is a chemical compound with the CAS Number: 33420-50-7 and a linear formula of C3H4F2O . It has a molecular weight of 94.06 .
Molecular Structure Analysis
The InChI code for 3,3-Difluorooxetane is 1S/C3H4F2O/c4-3(5)1-6-2-3/h1-2H2 . The compound has a molar refractivity of 15.9±0.4 cm^3 .Physical And Chemical Properties Analysis
3,3-Difluorooxetane has a density of 1.2±0.1 g/cm^3, a boiling point of 12.4±30.0 °C at 760 mmHg, and a vapour pressure of 1184.6±0.0 mmHg at 25°C . It has an enthalpy of vaporization of 24.9±3.0 kJ/mol and a flash point of -34.3±20.4 °C . The compound has an index of refraction of 1.337 .Scientific Research Applications
- Applications : It has been explored as a replacement for gem-dimethyl motifs, cyclic ketones, and morpholines in spirocyclic examples .
Peptidomimetics
Researchers have also investigated 3,3-difluorooxetane as an alternative isosteric replacement for amide carbonyls. For instance, 3-aminooxetanes have been used as peptidomimetics .
Chemical Synthesis and Novel Analogue Design
Understanding the effects of 3,3-difluorooxetanes in drug discovery settings is crucial. Chemical synthesis plays a significant role in driving novel analogue design. Notable examples include sulfoximines, bicyclo[1.1.1]pentane motifs, and oxetanes, each offering attractive physicochemical properties and increased 3-D character .
Future Directions
Oxetanes, including 3,3-Difluorooxetane, have gained significant interest in medicinal chemistry. They are seen as beneficial motifs because of their low molecular weight, high polarity, and three-dimensionality, which can improve properties including target affinity and aqueous solubility . Future directions may include further exploration of the properties of 3,3-Difluorooxetane and its potential applications in drug discovery .
Mechanism of Action
It’s worth noting that 3,3-difluorooxetane is a type of oxetane, a class of compounds that have received increasing interest in medicinal chemistry as attractive polar and low molecular weight motifs . Oxetanes have been demonstrated to often improve the chemical properties of target molecules for drug discovery purposes . They have been used as replacements for methylene, methyl, gem-dimethyl, and carbonyl groups .
The investigation of the properties of 3,3-diaryloxetanes, a related class of compounds, has shown that they can be used as potential bioisosteres, replacing problematic functionality while maintaining target activity and binding kinetics
properties
IUPAC Name |
3,3-difluorooxetane |
Source
|
---|---|---|
Details | Computed by LexiChem 2.6.6 (PubChem release 2019.06.18) | |
Source | PubChem | |
URL | https://pubchem.ncbi.nlm.nih.gov | |
Description | Data deposited in or computed by PubChem | |
InChI |
InChI=1S/C3H4F2O/c4-3(5)1-6-2-3/h1-2H2 |
Source
|
Details | Computed by InChI 1.0.5 (PubChem release 2019.06.18) | |
Source | PubChem | |
URL | https://pubchem.ncbi.nlm.nih.gov | |
Description | Data deposited in or computed by PubChem | |
InChI Key |
ZIJXRBOWCNYSSG-UHFFFAOYSA-N |
Source
|
Details | Computed by InChI 1.0.5 (PubChem release 2019.06.18) | |
Source | PubChem | |
URL | https://pubchem.ncbi.nlm.nih.gov | |
Description | Data deposited in or computed by PubChem | |
Canonical SMILES |
C1C(CO1)(F)F |
Source
|
Details | Computed by OEChem 2.1.5 (PubChem release 2019.06.18) | |
Source | PubChem | |
URL | https://pubchem.ncbi.nlm.nih.gov | |
Description | Data deposited in or computed by PubChem | |
Molecular Formula |
C3H4F2O |
Source
|
Details | Computed by PubChem 2.1 (PubChem release 2019.06.18) | |
Source | PubChem | |
URL | https://pubchem.ncbi.nlm.nih.gov | |
Description | Data deposited in or computed by PubChem | |
Molecular Weight |
94.06 g/mol |
Source
|
Details | Computed by PubChem 2.1 (PubChem release 2021.05.07) | |
Source | PubChem | |
URL | https://pubchem.ncbi.nlm.nih.gov | |
Description | Data deposited in or computed by PubChem | |
Product Name |
3,3-Difluorooxetane |
Disclaimer and Information on In-Vitro Research Products
Please be aware that all articles and product information presented on BenchChem are intended solely for informational purposes. The products available for purchase on BenchChem are specifically designed for in-vitro studies, which are conducted outside of living organisms. In-vitro studies, derived from the Latin term "in glass," involve experiments performed in controlled laboratory settings using cells or tissues. It is important to note that these products are not categorized as medicines or drugs, and they have not received approval from the FDA for the prevention, treatment, or cure of any medical condition, ailment, or disease. We must emphasize that any form of bodily introduction of these products into humans or animals is strictly prohibited by law. It is essential to adhere to these guidelines to ensure compliance with legal and ethical standards in research and experimentation.