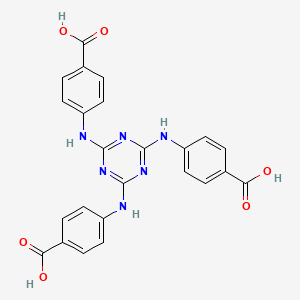
4,4',4''-((1,3,5-Triazine-2,4,6-triyl)tris(azanediyl))tribenzoic acid
- Click on QUICK INQUIRY to receive a quote from our team of experts.
- With the quality product at a COMPETITIVE price, you can focus more on your research.
Overview
Description
4,4',4''-((1,3,5-Triazine-2,4,6-triyl)tris(azanediyl))tribenzoic acid is a complex organic compound characterized by its triazine core and multiple carboxylic acid groups
Mechanism of Action
Target of Action
It is known that this compound can be used in the synthesis of an electrochromic material (ecm) . ECMs are materials that can change their optical properties (such as color) in response to an electric field .
Mode of Action
As an electron acceptor in the synthesis of ecms , it likely interacts with its targets by accepting electrons, which may result in changes to the optical properties of the ECM.
Biochemical Pathways
Given its role in the synthesis of ecms , it may influence pathways related to electron transfer and optical property modulation.
Result of Action
Its role as an electron acceptor in the synthesis of ecms suggests that it may contribute to changes in the optical properties of these materials.
Biochemical Analysis
Biochemical Properties
The compound 4,4’,4’'-((1,3,5-Triazine-2,4,6-triyl)tris(azanediyl))tribenzoic acid has been shown to interact with metal ions such as Cu2+ and Fe3+ . These interactions have been investigated at the liquid–solid interface by scanning tunneling microscopy (STM) . The modulation effects of these ions on the hydrogen-bonded structure of the compound have been observed .
Molecular Mechanism
The molecular mechanism of 4,4’,4’'-((1,3,5-Triazine-2,4,6-triyl)tris(azanediyl))tribenzoic acid involves coordination bonds with metal ions . For instance, a Cu2+ ion remains coordinated to two COOH groups of the compound, and this number doubles when the concentration of Cu2+ ions is doubled .
Temporal Effects in Laboratory Settings
The effects of 4,4’,4’'-((1,3,5-Triazine-2,4,6-triyl)tris(azanediyl))tribenzoic acid over time in laboratory settings have been observed through STM . The self-assembled structures of the compound formed by metal-ligand coordination bonds can be effectively adjusted by regulating the concentration of metal ions in a mixed solution .
Metabolic Pathways
Its ability to form coordination bonds with metal ions suggests potential involvement in metal-related metabolic pathways .
Transport and Distribution
Its interaction with metal ions suggests potential involvement with metal transporters or binding proteins .
Preparation Methods
Synthetic Routes and Reaction Conditions: The synthesis of 4,4',4''-((1,3,5-Triazine-2,4,6-triyl)tris(azanediyl))tribenzoic acid typically involves the reaction of 1,3,5-triazine with appropriate amines and carboxylic acids under controlled conditions. The reaction is often carried out in a solvent such as dimethylformamide (DMF) or dimethyl sulfoxide (DMSO) at elevated temperatures to facilitate the formation of the triazine ring.
Industrial Production Methods: In an industrial setting, the compound is produced through a multi-step synthesis process that includes the initial formation of the triazine core followed by the introduction of the carboxylic acid groups. The process requires precise control of reaction conditions, including temperature, pressure, and the use of catalysts to ensure high yield and purity.
Types of Reactions:
Oxidation: The carboxylic acid groups in the compound can undergo oxidation reactions to form carboxylate salts or other oxidized derivatives.
Reduction: Reduction reactions can be used to convert the carboxylic acid groups to alcohols or other reduced forms.
Common Reagents and Conditions:
Oxidation: Common oxidizing agents include potassium permanganate (KMnO₄) and chromium trioxide (CrO₃).
Reduction: Reducing agents such as lithium aluminum hydride (LiAlH₄) and sodium borohydride (NaBH₄) are often used.
Substitution: Various nucleophiles and electrophiles can be used to achieve substitution reactions, depending on the desired functional group.
Major Products Formed:
Oxidation: Carboxylate salts, ketones, and aldehydes.
Reduction: Alcohols and other reduced derivatives.
Substitution: Derivatives with different functional groups attached to the triazine ring.
Scientific Research Applications
Chemistry: In chemistry, 4,4',4''-((1,3,5-Triazine-2,4,6-triyl)tris(azanediyl))tribenzoic acid is used as a building block for the synthesis of more complex molecules. Its ability to form stable structures makes it valuable in the design of new materials and catalysts.
Biology: The compound has potential applications in biological research, particularly in the study of cellular processes and the development of new drugs. Its structural similarity to certain biological molecules allows it to interact with biological targets, making it useful in drug discovery and development.
Medicine: In medicine, the compound can be used as a precursor for the synthesis of pharmaceuticals. Its carboxylic acid groups can be modified to create active pharmaceutical ingredients (APIs) with specific therapeutic properties.
Industry: In industry, this compound is used in the production of advanced materials, such as electrochromic materials for smart windows and electronic paper. Its ability to undergo redox reactions makes it suitable for applications in energy storage devices.
Comparison with Similar Compounds
4,4',4''-((1,3,5-Triazine-2,4,6-triyl)trianiline)
4,4',4''-((1,3,5-Triazine-2,4,6-triyl)tris(aminomethyl))benzoic acid
4,4',4''-((1,3,5-Triazine-2,4,6-triyl)tris(ethanediyl))tribenzoic acid
Uniqueness: 4,4',4''-((1,3,5-Triazine-2,4,6-triyl)tris(azanediyl))tribenzoic acid is unique due to its combination of triazine core and multiple carboxylic acid groups. This structural feature allows for a wide range of chemical reactions and applications, making it more versatile than similar compounds.
Properties
IUPAC Name |
4-[[4,6-bis(4-carboxyanilino)-1,3,5-triazin-2-yl]amino]benzoic acid |
Source
|
---|---|---|
Details | Computed by Lexichem TK 2.7.0 (PubChem release 2021.05.07) | |
Source | PubChem | |
URL | https://pubchem.ncbi.nlm.nih.gov | |
Description | Data deposited in or computed by PubChem | |
InChI |
InChI=1S/C24H18N6O6/c31-19(32)13-1-7-16(8-2-13)25-22-28-23(26-17-9-3-14(4-10-17)20(33)34)30-24(29-22)27-18-11-5-15(6-12-18)21(35)36/h1-12H,(H,31,32)(H,33,34)(H,35,36)(H3,25,26,27,28,29,30) |
Source
|
Details | Computed by InChI 1.0.6 (PubChem release 2021.05.07) | |
Source | PubChem | |
URL | https://pubchem.ncbi.nlm.nih.gov | |
Description | Data deposited in or computed by PubChem | |
InChI Key |
NGJJZCPADSICRI-UHFFFAOYSA-N |
Source
|
Details | Computed by InChI 1.0.6 (PubChem release 2021.05.07) | |
Source | PubChem | |
URL | https://pubchem.ncbi.nlm.nih.gov | |
Description | Data deposited in or computed by PubChem | |
Canonical SMILES |
C1=CC(=CC=C1C(=O)O)NC2=NC(=NC(=N2)NC3=CC=C(C=C3)C(=O)O)NC4=CC=C(C=C4)C(=O)O |
Source
|
Details | Computed by OEChem 2.3.0 (PubChem release 2021.05.07) | |
Source | PubChem | |
URL | https://pubchem.ncbi.nlm.nih.gov | |
Description | Data deposited in or computed by PubChem | |
Molecular Formula |
C24H18N6O6 |
Source
|
Details | Computed by PubChem 2.1 (PubChem release 2021.05.07) | |
Source | PubChem | |
URL | https://pubchem.ncbi.nlm.nih.gov | |
Description | Data deposited in or computed by PubChem | |
Molecular Weight |
486.4 g/mol |
Source
|
Details | Computed by PubChem 2.1 (PubChem release 2021.05.07) | |
Source | PubChem | |
URL | https://pubchem.ncbi.nlm.nih.gov | |
Description | Data deposited in or computed by PubChem | |
Disclaimer and Information on In-Vitro Research Products
Please be aware that all articles and product information presented on BenchChem are intended solely for informational purposes. The products available for purchase on BenchChem are specifically designed for in-vitro studies, which are conducted outside of living organisms. In-vitro studies, derived from the Latin term "in glass," involve experiments performed in controlled laboratory settings using cells or tissues. It is important to note that these products are not categorized as medicines or drugs, and they have not received approval from the FDA for the prevention, treatment, or cure of any medical condition, ailment, or disease. We must emphasize that any form of bodily introduction of these products into humans or animals is strictly prohibited by law. It is essential to adhere to these guidelines to ensure compliance with legal and ethical standards in research and experimentation.