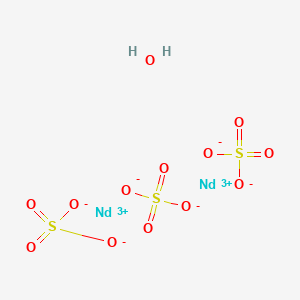
Neodymium(III) sulfate hydrate
Overview
Description
Neodymium(III) sulfate hydrate is a chemical compound consisting of neodymium, a rare-earth metal, and sulfate ions. It is commonly found in its hydrated form, with the octahydrate being the most prevalent. This compound is known for its pink crystalline appearance and has a chemical formula of Nd₂(SO₄)₃·xH₂O. This compound is notable for its retrograde solubility, meaning its solubility decreases with increasing temperature .
Scientific Research Applications
Neodymium(III) sulfate hydrate has a wide range of applications in scientific research and industry:
Chemistry: It is used in the study of rare-earth element interactions and as a precursor for other neodymium compounds.
Biology and Medicine: While not commonly used directly in biological systems, its derivatives are explored for potential medical applications.
Industry: It is utilized in the production of powerful lasers, glass coloring, and tinting.
Mechanism of Action
Target of Action
Neodymium(III) sulfate hydrate is a salt of the rare-earth metal neodymium . The primary targets of this compound are the rare earth elements (REEs) in various resources such as ore concentrates, recycled permanent Nd-Fe-B magnets, and rare earth powder waste . These REEs are critical to numerous industries, including high technology devices .
Mode of Action
The interaction of Neodymium(III) with sulfate at high ionic strengths is crucial for the extraction process . In a standard process, concentrated sulfuric acid (H2SO4) is used as an extraction/leaching agent . The thermodynamic model developed can describe the interaction of Neodymium(III) with sulfate to ionic strengths up to 16.5 mol·kg –1 and to temperatures up to 100 °C .
Biochemical Pathways
The biochemical pathways affected by this compound primarily involve the extraction and recovery of REEs from various resources . The extraction process involves roasting the resources with H2SO4 at elevated temperatures . The thermodynamic model can be used to design and optimize this chemical process .
Result of Action
The result of the action of this compound is the successful extraction of REEs from various resources . This extraction process is crucial for numerous industries that rely on these elements .
Action Environment
The action of this compound is influenced by environmental factors such as ionic strength and temperature . Its interaction with sulfate can be described up to ionic strengths of 16.5 mol·kg –1 and temperatures up to 100 °C . Furthermore, the solubility of this compound decreases with increasing temperature , which can also influence its action, efficacy, and stability.
Safety and Hazards
Neodymium(III) sulfate hydrate can cause skin irritation and serious eye irritation . It may also cause respiratory irritation . It is recommended to avoid breathing dust, gas, or vapors, avoid contacting with skin and eye, use personal protective equipment, wear chemical impermeable gloves, ensure adequate ventilation, remove all sources of ignition, evacuate personnel to safe areas, and keep people away from and upwind of spill/leak .
Relevant Papers A paper titled “A Thermodynamic Model for Nd(III)–Sulfate Interaction at High Ionic Strengths and Elevated Temperatures: Applications to Rare Earth Element Extraction” provides valuable insights into the extraction process of Neodymium and its interaction with sulfate .
Preparation Methods
Synthetic Routes and Reaction Conditions: Neodymium(III) sulfate hydrate can be synthesized through the reaction of neodymium(III) oxide with sulfuric acid: [ \text{Nd}_2\text{O}_3 + 3\text{H}_2\text{SO}_4 \rightarrow \text{Nd}_2(\text{SO}_4)_3 + 3\text{H}_2\text{O} ] Alternatively, it can be prepared by reacting neodymium(III) perchlorate with sodium sulfate .
Industrial Production Methods: In industrial settings, neodymium is often extracted from ore concentrates, recycled materials, or waste products using concentrated sulfuric acid as an extraction agent. This process involves dissolving neodymium-containing materials in sulfuric acid to produce neodymium(III) sulfate .
Chemical Reactions Analysis
Types of Reactions: Neodymium(III) sulfate hydrate primarily undergoes decomposition reactions. For instance, the octahydrate form decomposes to the pentahydrate at 40°C, which further decomposes to the dihydrate at 145°C. The dihydrate then dehydrates to the anhydrous form at 290°C .
Common Reagents and Conditions:
Oxidation and Reduction: this compound does not typically undergo oxidation or reduction reactions under standard conditions.
Substitution: It can participate in substitution reactions where sulfate ions are replaced by other anions, such as in the reaction with sodium perchlorate.
Major Products:
Decomposition Products: Pentahydrate, dihydrate, and anhydrous neodymium(III) sulfate.
Substitution Products: Neodymium(III) perchlorate when reacted with sodium perchlorate.
Comparison with Similar Compounds
Neodymium(III) nitrate: Similar in that it is also a neodymium salt, but it is formed with nitrate ions instead of sulfate.
Neodymium(III) chloride: Another neodymium salt, formed with chloride ions.
Praseodymium(III) sulfate: A similar rare-earth sulfate compound, but with praseodymium instead of neodymium.
Uniqueness: Neodymium(III) sulfate hydrate is unique due to its retrograde solubility and its specific applications in laser technology and glass coloring. Its ability to form multiple hydrates also distinguishes it from other neodymium compounds .
Properties
IUPAC Name |
neodymium(3+);trisulfate;hydrate | |
---|---|---|
Details | Computed by Lexichem TK 2.7.0 (PubChem release 2021.05.07) | |
Source | PubChem | |
URL | https://pubchem.ncbi.nlm.nih.gov | |
Description | Data deposited in or computed by PubChem | |
InChI |
InChI=1S/2Nd.3H2O4S.H2O/c;;3*1-5(2,3)4;/h;;3*(H2,1,2,3,4);1H2/q2*+3;;;;/p-6 | |
Details | Computed by InChI 1.0.6 (PubChem release 2021.05.07) | |
Source | PubChem | |
URL | https://pubchem.ncbi.nlm.nih.gov | |
Description | Data deposited in or computed by PubChem | |
InChI Key |
RHVPCSSKNPYQDU-UHFFFAOYSA-H | |
Details | Computed by InChI 1.0.6 (PubChem release 2021.05.07) | |
Source | PubChem | |
URL | https://pubchem.ncbi.nlm.nih.gov | |
Description | Data deposited in or computed by PubChem | |
Canonical SMILES |
O.[O-]S(=O)(=O)[O-].[O-]S(=O)(=O)[O-].[O-]S(=O)(=O)[O-].[Nd+3].[Nd+3] | |
Details | Computed by OEChem 2.3.0 (PubChem release 2021.05.07) | |
Source | PubChem | |
URL | https://pubchem.ncbi.nlm.nih.gov | |
Description | Data deposited in or computed by PubChem | |
Molecular Formula |
H2Nd2O13S3 | |
Details | Computed by PubChem 2.1 (PubChem release 2021.05.07) | |
Source | PubChem | |
URL | https://pubchem.ncbi.nlm.nih.gov | |
Description | Data deposited in or computed by PubChem | |
DSSTOX Substance ID |
DTXSID80692902 | |
Record name | Neodymium sulfate--water (2/3/1) | |
Source | EPA DSSTox | |
URL | https://comptox.epa.gov/dashboard/DTXSID80692902 | |
Description | DSSTox provides a high quality public chemistry resource for supporting improved predictive toxicology. | |
Molecular Weight |
594.7 g/mol | |
Details | Computed by PubChem 2.1 (PubChem release 2021.05.07) | |
Source | PubChem | |
URL | https://pubchem.ncbi.nlm.nih.gov | |
Description | Data deposited in or computed by PubChem | |
CAS No. |
101509-27-7 | |
Record name | Neodymium sulfate--water (2/3/1) | |
Source | EPA DSSTox | |
URL | https://comptox.epa.gov/dashboard/DTXSID80692902 | |
Description | DSSTox provides a high quality public chemistry resource for supporting improved predictive toxicology. | |
Disclaimer and Information on In-Vitro Research Products
Please be aware that all articles and product information presented on BenchChem are intended solely for informational purposes. The products available for purchase on BenchChem are specifically designed for in-vitro studies, which are conducted outside of living organisms. In-vitro studies, derived from the Latin term "in glass," involve experiments performed in controlled laboratory settings using cells or tissues. It is important to note that these products are not categorized as medicines or drugs, and they have not received approval from the FDA for the prevention, treatment, or cure of any medical condition, ailment, or disease. We must emphasize that any form of bodily introduction of these products into humans or animals is strictly prohibited by law. It is essential to adhere to these guidelines to ensure compliance with legal and ethical standards in research and experimentation.