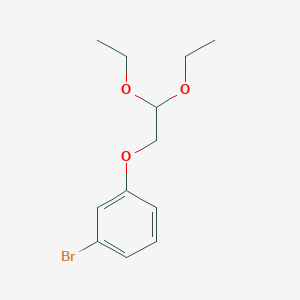
1-Bromo-3-(2,2-diethoxyethoxy)benzene
Overview
Description
“1-Bromo-3-(2,2-diethoxyethoxy)benzene” is a chemical compound with the molecular formula C12H17BrO3 . It is provided by Sigma-Aldrich to early discovery researchers as part of a collection of unique chemicals .
Molecular Structure Analysis
The molecular structure of “1-Bromo-3-(2,2-diethoxyethoxy)benzene” consists of a benzene ring with a bromine atom and a 2,2-diethoxyethoxy group attached to it . The exact mass of the molecule is 288.03600 .Physical And Chemical Properties Analysis
The physical and chemical properties of “1-Bromo-3-(2,2-diethoxyethoxy)benzene” include a molecular weight of 289.16600 and a LogP value of 3.22700 . Unfortunately, other properties like density, boiling point, and melting point are not available .Scientific Research Applications
A Facile Synthesis of Isoindoles A two-step synthesis of 1-substituted 3-alkoxy-1H-isoindoles has been developed, involving the reaction of 2-(dialkoxymethyl)phenyllithium compounds (generated via Br/Li exchange between 1-bromo-2-(dialkoxymethyl)benzenes and BuLi) with nitriles, followed by an acid-catalyzed cyclization. This process demonstrates the versatility of 1-bromo-2-(dialkoxymethyl)benzenes in organic synthesis, particularly in the construction of isoindole frameworks (Kuroda & Kobayashi, 2015).
Synthesis of Chiral 1-Halo-1-Bromo Compounds The reaction of 1,2-halohydrins (derived from carbohydrates) with (diacetoxyiodo)benzene and bromine is a method to synthesize 1-deoxy-1-halo-1-bromo-alditols. This pathway involves β-fragmentation of the intermediate anomeric alkoxyl radicals and highlights the role of brominated intermediates in the synthesis of complex organic molecules (González et al., 2003).
Applications in Molecular Electronics Aryl bromides, such as 4-bromophenyl tert-butyl sulfide and 1-bromo-4-(methoxymethyl)benzene, are essential building blocks for molecular wires used in molecular electronics. These compounds undergo efficient transformations to form oligo(phenylenevinylene) and oligo(phenyleneethynylene) wires, illustrating the significance of brominated compounds in advanced material sciences (Stuhr-Hansen et al., 2005).
properties
IUPAC Name |
1-bromo-3-(2,2-diethoxyethoxy)benzene | |
---|---|---|
Details | Computed by LexiChem 2.6.6 (PubChem release 2019.06.18) | |
Source | PubChem | |
URL | https://pubchem.ncbi.nlm.nih.gov | |
Description | Data deposited in or computed by PubChem | |
InChI |
InChI=1S/C12H17BrO3/c1-3-14-12(15-4-2)9-16-11-7-5-6-10(13)8-11/h5-8,12H,3-4,9H2,1-2H3 | |
Details | Computed by InChI 1.0.5 (PubChem release 2019.06.18) | |
Source | PubChem | |
URL | https://pubchem.ncbi.nlm.nih.gov | |
Description | Data deposited in or computed by PubChem | |
InChI Key |
BXNLYIJBCFCSBQ-UHFFFAOYSA-N | |
Details | Computed by InChI 1.0.5 (PubChem release 2019.06.18) | |
Source | PubChem | |
URL | https://pubchem.ncbi.nlm.nih.gov | |
Description | Data deposited in or computed by PubChem | |
Canonical SMILES |
CCOC(COC1=CC(=CC=C1)Br)OCC | |
Details | Computed by OEChem 2.1.5 (PubChem release 2019.06.18) | |
Source | PubChem | |
URL | https://pubchem.ncbi.nlm.nih.gov | |
Description | Data deposited in or computed by PubChem | |
Molecular Formula |
C12H17BrO3 | |
Details | Computed by PubChem 2.1 (PubChem release 2019.06.18) | |
Source | PubChem | |
URL | https://pubchem.ncbi.nlm.nih.gov | |
Description | Data deposited in or computed by PubChem | |
Molecular Weight |
289.16 g/mol | |
Details | Computed by PubChem 2.1 (PubChem release 2021.05.07) | |
Source | PubChem | |
URL | https://pubchem.ncbi.nlm.nih.gov | |
Description | Data deposited in or computed by PubChem | |
Product Name |
1-Bromo-3-(2,2-diethoxyethoxy)benzene |
Synthesis routes and methods I
Procedure details
Synthesis routes and methods II
Procedure details
Synthesis routes and methods III
Procedure details
Disclaimer and Information on In-Vitro Research Products
Please be aware that all articles and product information presented on BenchChem are intended solely for informational purposes. The products available for purchase on BenchChem are specifically designed for in-vitro studies, which are conducted outside of living organisms. In-vitro studies, derived from the Latin term "in glass," involve experiments performed in controlled laboratory settings using cells or tissues. It is important to note that these products are not categorized as medicines or drugs, and they have not received approval from the FDA for the prevention, treatment, or cure of any medical condition, ailment, or disease. We must emphasize that any form of bodily introduction of these products into humans or animals is strictly prohibited by law. It is essential to adhere to these guidelines to ensure compliance with legal and ethical standards in research and experimentation.