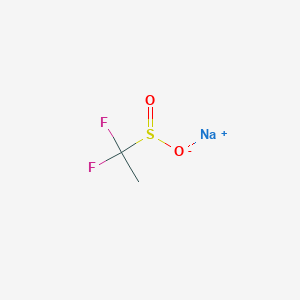
Sodium 1,1-difluoroethanesulfinate
- Click on QUICK INQUIRY to receive a quote from our team of experts.
- With the quality product at a COMPETITIVE price, you can focus more on your research.
Overview
Description
Sodium 1,1-difluoroethanesulfinate is a chemical compound with the empirical formula C2H3F2NaO2S and a molecular weight of 152.10 g/mol . It is commonly used in organic synthesis, particularly for the direct alkylation of heterocycles. This compound is known for its ability to introduce a 1,1-difluoroethyl group into various substrates, making it a valuable reagent in the field of fluorine chemistry .
Mechanism of Action
Target of Action
Sodium 1,1-difluoroethanesulfinate is primarily used as a reagent in chemical reactions . Its primary targets are organic compounds, specifically heterocycles . The role of this compound is to facilitate the direct alkylation of these heterocycles .
Mode of Action
The this compound interacts with its targets by appending a 1,1-difluoroethyl group . This interaction results in the formation of fluorinated heteroarylether bioisosteres .
Biochemical Pathways
The biochemical pathway affected by this compound involves the direct alkylation of heterocycles . The downstream effects of this pathway include the creation of fluorinated heteroarylether bioisosteres .
Result of Action
The molecular effect of this compound’s action is the formation of a 1,1-difluoroethyl group . On a cellular level, this can lead to the creation of fluorinated heteroarylether bioisosteres .
Biochemical Analysis
Biochemical Properties
Sodium 1,1-difluoroethanesulfinate plays a significant role in biochemical reactions, particularly in the formation of fluorinated heteroarylether bioisosteres . This interaction is crucial for the diversification of chemical structures and the prediction of metabolic pathways . The compound’s ability to act as a catalyst in C-C bond formation and fluorination reactions highlights its importance in biochemical research .
Cellular Effects
This compound has been shown to influence various cellular processes. It affects cell signaling pathways, gene expression, and cellular metabolism . The compound’s interaction with cellular proteins can lead to changes in the activity of specific enzymes, thereby altering metabolic flux and the levels of certain metabolites . These effects are crucial for understanding the compound’s potential therapeutic applications and its impact on cellular function.
Molecular Mechanism
The molecular mechanism of action of this compound involves its binding interactions with biomolecules . The compound can inhibit or activate specific enzymes, leading to changes in gene expression and cellular metabolism . Its ability to append a 1,1-difluoroethyl group to target molecules is facilitated by its interaction with specific proteins and enzymes
Temporal Effects in Laboratory Settings
In laboratory settings, the effects of this compound can change over time. The compound’s stability and degradation are critical factors that influence its long-term effects on cellular function . Studies have shown that the compound remains stable under specific conditions, allowing for its prolonged use in biochemical experiments . Its degradation over time can lead to changes in its efficacy and impact on cellular processes .
Dosage Effects in Animal Models
The effects of this compound vary with different dosages in animal models. At lower doses, the compound may exhibit minimal toxicity and adverse effects . At higher doses, it can lead to toxic effects and significant changes in cellular function . Understanding the dosage effects is crucial for determining the compound’s therapeutic potential and its safety profile in preclinical studies .
Metabolic Pathways
This compound is involved in various metabolic pathways, interacting with specific enzymes and cofactors . Its role in the formation of fluorinated heteroarylether bioisosteres highlights its importance in metabolic flux and the regulation of metabolite levels .
Transport and Distribution
The transport and distribution of this compound within cells and tissues are influenced by its interaction with specific transporters and binding proteins . These interactions determine the compound’s localization and accumulation in different cellular compartments . Understanding the transport and distribution mechanisms is crucial for determining the compound’s efficacy and potential therapeutic applications .
Subcellular Localization
This compound’s subcellular localization is influenced by targeting signals and post-translational modifications . These factors direct the compound to specific compartments or organelles, affecting its activity and function .
Preparation Methods
The synthesis of sodium 1,1-difluoroethanesulfinate typically involves the use of Hu’s reagent as a starting material. The synthetic route includes alkylation with methyl iodide, followed by cleavage and liberation of the sodium sulfinate salt in the presence of sodium hydride and ethyl mercaptan . This method provides a straightforward and efficient approach to obtaining the compound.
Industrial production methods for this compound are not extensively documented, but they likely follow similar synthetic routes with optimizations for large-scale production.
Chemical Reactions Analysis
Sodium 1,1-difluoroethanesulfinate undergoes various types of chemical reactions, including:
Substitution Reactions: It can participate in nucleophilic substitution reactions, where the 1,1-difluoroethyl group is introduced into different substrates.
Oxidation and Reduction Reactions:
C-C Bond Formation: This compound is particularly useful in forming carbon-carbon bonds through reactions such as fluorinations and C-H activation.
Common reagents and conditions used in these reactions include catalysts, solvents, and specific temperature and pressure conditions tailored to the desired transformation. The major products formed from these reactions typically include fluorinated heteroarylether bioisosteres .
Scientific Research Applications
Sodium 1,1-difluoroethanesulfinate has a wide range of applications in scientific research:
Biology and Medicine: The compound’s ability to modify bioactive molecules makes it valuable in drug discovery and development, particularly for creating fluorinated analogs of pharmaceuticals.
Comparison with Similar Compounds
Sodium 1,1-difluoroethanesulfinate can be compared with other similar compounds, such as:
Sodium triflinate: Used for similar fluorination reactions but introduces a trifluoromethyl group instead of a difluoroethyl group.
Zinc difluoromethanesulfinate: Another reagent for introducing difluoromethyl groups, but with different reactivity and applications.
Sodium 1-(trifluoromethyl)cyclopropanesulfinate:
The uniqueness of this compound lies in its ability to introduce a 1,1-difluoroethyl group, which can impart distinct chemical and physical properties to the resulting compounds .
Properties
IUPAC Name |
sodium;1,1-difluoroethanesulfinate |
Source
|
---|---|---|
Details | Computed by LexiChem 2.6.6 (PubChem release 2019.06.18) | |
Source | PubChem | |
URL | https://pubchem.ncbi.nlm.nih.gov | |
Description | Data deposited in or computed by PubChem | |
InChI |
InChI=1S/C2H4F2O2S.Na/c1-2(3,4)7(5)6;/h1H3,(H,5,6);/q;+1/p-1 |
Source
|
Details | Computed by InChI 1.0.5 (PubChem release 2019.06.18) | |
Source | PubChem | |
URL | https://pubchem.ncbi.nlm.nih.gov | |
Description | Data deposited in or computed by PubChem | |
InChI Key |
YAUVUSSCQUHHFC-UHFFFAOYSA-M |
Source
|
Details | Computed by InChI 1.0.5 (PubChem release 2019.06.18) | |
Source | PubChem | |
URL | https://pubchem.ncbi.nlm.nih.gov | |
Description | Data deposited in or computed by PubChem | |
Canonical SMILES |
CC(F)(F)S(=O)[O-].[Na+] |
Source
|
Details | Computed by OEChem 2.1.5 (PubChem release 2019.06.18) | |
Source | PubChem | |
URL | https://pubchem.ncbi.nlm.nih.gov | |
Description | Data deposited in or computed by PubChem | |
Molecular Formula |
C2H3F2NaO2S |
Source
|
Details | Computed by PubChem 2.1 (PubChem release 2019.06.18) | |
Source | PubChem | |
URL | https://pubchem.ncbi.nlm.nih.gov | |
Description | Data deposited in or computed by PubChem | |
Molecular Weight |
152.10 g/mol |
Source
|
Details | Computed by PubChem 2.1 (PubChem release 2021.05.07) | |
Source | PubChem | |
URL | https://pubchem.ncbi.nlm.nih.gov | |
Description | Data deposited in or computed by PubChem | |
CAS No. |
1422738-67-7 |
Source
|
Record name | sodium 1,1-difluoroethane-1-sulfinate | |
Source | European Chemicals Agency (ECHA) | |
URL | https://echa.europa.eu/information-on-chemicals | |
Description | The European Chemicals Agency (ECHA) is an agency of the European Union which is the driving force among regulatory authorities in implementing the EU's groundbreaking chemicals legislation for the benefit of human health and the environment as well as for innovation and competitiveness. | |
Explanation | Use of the information, documents and data from the ECHA website is subject to the terms and conditions of this Legal Notice, and subject to other binding limitations provided for under applicable law, the information, documents and data made available on the ECHA website may be reproduced, distributed and/or used, totally or in part, for non-commercial purposes provided that ECHA is acknowledged as the source: "Source: European Chemicals Agency, http://echa.europa.eu/". Such acknowledgement must be included in each copy of the material. ECHA permits and encourages organisations and individuals to create links to the ECHA website under the following cumulative conditions: Links can only be made to webpages that provide a link to the Legal Notice page. | |
Disclaimer and Information on In-Vitro Research Products
Please be aware that all articles and product information presented on BenchChem are intended solely for informational purposes. The products available for purchase on BenchChem are specifically designed for in-vitro studies, which are conducted outside of living organisms. In-vitro studies, derived from the Latin term "in glass," involve experiments performed in controlled laboratory settings using cells or tissues. It is important to note that these products are not categorized as medicines or drugs, and they have not received approval from the FDA for the prevention, treatment, or cure of any medical condition, ailment, or disease. We must emphasize that any form of bodily introduction of these products into humans or animals is strictly prohibited by law. It is essential to adhere to these guidelines to ensure compliance with legal and ethical standards in research and experimentation.