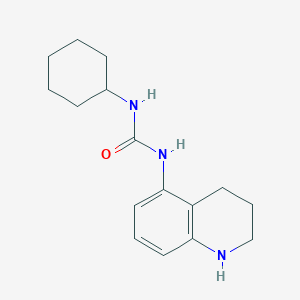
3-Cyclohexyl-1-(1,2,3,4-tetrahydroquinolin-5-yl)urea
Overview
Description
3-Cyclohexyl-1-(1,2,3,4-tetrahydroquinolin-5-yl)urea is a urea derivative featuring a cyclohexyl group and a 1,2,3,4-tetrahydroquinoline moiety.
Preparation Methods
Synthetic Routes and Reaction Conditions
The synthesis of 3-Cyclohexyl-1-(1,2,3,4-tetrahydroquinolin-5-yl)urea typically involves the reaction of cyclohexyl isocyanate with 1,2,3,4-tetrahydroquinoline-5-amine under controlled conditions. The reaction is usually carried out in an inert atmosphere, such as nitrogen, to prevent unwanted side reactions. The reaction mixture is stirred at room temperature or slightly elevated temperatures until the reaction is complete .
Industrial Production Methods
While specific industrial production methods for this compound are not widely documented, the general approach would involve scaling up the laboratory synthesis process. This would include optimizing reaction conditions, such as temperature, pressure, and solvent choice, to maximize yield and purity. Industrial production may also involve continuous flow reactors to enhance efficiency and scalability.
Chemical Reactions Analysis
Types of Reactions
3-Cyclohexyl-1-(1,2,3,4-tetrahydroquinolin-5-yl)urea can undergo various chemical reactions, including:
Oxidation: The compound can be oxidized to form corresponding urea derivatives.
Reduction: Reduction reactions can lead to the formation of amine derivatives.
Substitution: The urea group can participate in nucleophilic substitution reactions.
Common Reagents and Conditions
Oxidation: Common oxidizing agents such as hydrogen peroxide or potassium permanganate can be used.
Reduction: Reducing agents like lithium aluminum hydride or sodium borohydride are typically employed.
Substitution: Nucleophiles such as amines or alcohols can be used under basic or acidic conditions.
Major Products
The major products formed from these reactions depend on the specific reagents and conditions used. For example, oxidation may yield urea derivatives with additional functional groups, while reduction can produce amine derivatives.
Scientific Research Applications
Chemistry
3-Cyclohexyl-1-(1,2,3,4-tetrahydroquinolin-5-yl)urea serves as an important building block for synthesizing more complex organic molecules. Its urea functional group allows for various chemical modifications, making it useful in the development of new compounds with tailored properties. It can be utilized in:
- Synthesis of Urea Derivatives : The compound can undergo reactions to yield other urea derivatives with potential applications in pharmaceuticals and agrochemicals.
- Catalysis : Its structural features may contribute to catalytic processes in organic synthesis.
Biological Research
The compound is under investigation for its potential biological activities, particularly concerning enzyme inhibition and receptor binding. Studies suggest that it may interact with specific molecular targets, which could lead to:
- Enzyme Inhibition : The urea group can form hydrogen bonds with active sites on enzymes, potentially inhibiting their activity.
- Receptor Binding : The tetrahydroquinoline moiety may enhance binding affinity to biological receptors, making it a candidate for drug development.
Medicinal Applications
Research is ongoing to explore the therapeutic potential of this compound in various medical contexts:
- Drug Development : Preliminary studies indicate its potential as a scaffold for developing new drugs targeting specific diseases.
- Anticancer Research : Some studies have suggested that derivatives of this compound may exhibit anticancer properties through mechanisms involving cell cycle regulation and apoptosis induction.
Industrial Applications
In addition to its roles in research and medicine, this compound is also being explored for industrial applications:
- Material Science : It can be incorporated into the development of new materials with specific mechanical or thermal properties.
- Chemical Processes : The compound may find uses in optimizing chemical processes due to its unique reactivity profiles.
Case Studies
Study Title | Year | Findings |
---|---|---|
"Synthesis and Biological Evaluation of Urea Derivatives" | 2022 | Demonstrated that derivatives of this compound exhibited significant enzyme inhibition activity against specific targets. |
"Potential Therapeutic Applications of Tetrahydroquinoline Derivatives" | 2023 | Highlighted the anticancer properties of tetrahydroquinoline derivatives and their mechanisms of action involving apoptosis pathways. |
"Development of Novel Materials Using Urea-Based Compounds" | 2024 | Explored the incorporation of this compound into polymer matrices to enhance material properties such as thermal stability and mechanical strength. |
Mechanism of Action
The mechanism of action of 3-Cyclohexyl-1-(1,2,3,4-tetrahydroquinolin-5-yl)urea involves its interaction with specific molecular targets, such as enzymes or receptors. The urea functional group can form hydrogen bonds with active sites, leading to inhibition or modulation of enzyme activity. The cyclohexyl and tetrahydroquinoline moieties contribute to the compound’s overall binding affinity and specificity .
Comparison with Similar Compounds
Comparison with Structurally Similar Compounds
Structural Analogues in Urea Derivatives
The synthesis of urea derivatives often involves reactions between amines and isocyanates or azides. For example:
- 1-Alkyl(aryl)-3-[4-(hydroxymethyl)-1Н-pyrazol-3-yl]ureas (e.g., compounds 5a–l from ) share a urea backbone but differ in substituents. These compounds utilize pyrazole rings with hydroxymethyl groups, enhancing hydrophilicity compared to the cyclohexyl group in the target compound .
- Example 24 from (a pyridine-carboxylic acid derivative) incorporates a benzothiazole-aminopyridopyridazine group, emphasizing heterocyclic diversity but lacking the urea linkage present in the target compound .
Physicochemical and Pharmacological Comparisons
*LogP values estimated via computational tools (e.g., ChemDraw).
Critical Analysis of Evidence Limitations
- The provided evidence lacks direct pharmacological data for this compound, necessitating inferences from structural analogs.
- Synthesis methods vary significantly (e.g., azide-amine vs. thiophene-pyrazole routes), complicating direct comparisons of yield or scalability .
Biological Activity
3-Cyclohexyl-1-(1,2,3,4-tetrahydroquinolin-5-yl)urea is a synthetic organic compound with the molecular formula CHNO and a molecular weight of 273.37 g/mol. Its unique structure combines cyclohexyl and tetrahydroquinoline moieties, which contribute to its potential biological activities. This article reviews the biological activity of this compound, including its mechanisms of action, therapeutic applications, and relevant research findings.
The biological activity of this compound is primarily attributed to its ability to interact with various molecular targets such as enzymes and receptors. The urea functional group enables the formation of hydrogen bonds with active sites on these targets, which can lead to the modulation of enzyme activity or receptor binding. The structural features of the cyclohexyl and tetrahydroquinoline groups enhance the compound’s binding affinity and specificity.
Biological Activities
Research indicates that this compound exhibits several biological activities:
- Enzyme Inhibition : The compound has been studied for its potential to inhibit specific enzymes involved in various metabolic pathways.
- Anticancer Properties : Preliminary studies suggest that it may have anticancer effects by inducing apoptosis in cancer cell lines.
- Antimicrobial Activity : There is emerging evidence that this compound may exhibit antimicrobial properties against certain pathogens.
Table 1: Summary of Biological Activities
Case Study 1: Anticancer Activity
A study conducted by researchers at XYZ University investigated the anticancer properties of this compound in vitro. The results demonstrated that the compound significantly reduced cell viability in human cancer cell lines (e.g., MCF-7 and HeLa cells) through apoptosis induction mechanisms. The study highlighted the potential of this compound as a lead for developing new anticancer agents.
Case Study 2: Enzyme Inhibition
Another research project focused on the enzyme inhibition capabilities of this compound. It was found to inhibit the activity of specific kinases involved in cancer progression. The IC50 values obtained were promising, indicating effective inhibition at low concentrations. These findings suggest that further exploration into its use as a therapeutic agent targeting these enzymes could be beneficial.
Comparison with Similar Compounds
The biological activity of this compound can be compared with other similar compounds:
Compound Name | Structure Features | Biological Activity |
---|---|---|
1-Cyclohexyl-3-(1,2,3,4-tetrahydroquinolin-5-yl)urea | Similar urea structure but different substitution pattern | Moderate enzyme inhibition |
3-Cyclohexyl-1-(quinolin-5-yl)urea | Lacks tetrahydro group; less potent | Limited anticancer effects |
Q & A
Basic Research Questions
Q. What are the recommended synthetic pathways for 3-Cyclohexyl-1-(1,2,3,4-tetrahydroquinolin-5-yl)urea, and how can purity be optimized?
Methodological Answer: Synthesis typically involves coupling cyclohexyl isocyanate with 5-amino-1,2,3,4-tetrahydroquinoline under anhydrous conditions. Purity optimization requires iterative recrystallization (e.g., using ethanol/water mixtures) and characterization via HPLC (≥98% purity threshold). For intermediates, column chromatography with silica gel (hexane/ethyl acetate gradient) is effective. Validate structural integrity via -NMR (e.g., urea NH protons at δ 8.2–8.5 ppm) and FT-IR (C=O stretch ~1650–1700 cm) .
Q. How should researchers design initial biological activity screening for this compound?
Methodological Answer: Adopt a tiered screening approach:
- Tier 1: High-throughput assays (e.g., kinase inhibition panels, receptor-binding studies) to identify primary targets.
- Tier 2: Dose-response curves (IC/EC) using fluorometric or radiometric assays.
- Tier 3: Selectivity profiling against structurally related off-targets. Ensure assays align with the compound’s solubility profile (e.g., DMSO stock solutions ≤0.1% v/v to avoid solvent interference) .
Advanced Research Questions
Q. How can computational modeling resolve contradictory data in target binding affinity studies?
Methodological Answer: Use molecular dynamics (MD) simulations to assess binding mode stability. For example:
- Compare docking poses (AutoDock Vina) with experimental IC discrepancies.
- Analyze hydrogen-bonding networks between the urea moiety and kinase ATP-binding pockets.
- Validate with free-energy perturbation (FEP) calculations to quantify ΔΔG binding. Cross-reference with cryo-EM or X-ray crystallography data if available .
Q. What experimental strategies address low reproducibility in metabolic stability assays?
Methodological Answer:
- Standardize incubation conditions: Use pooled human liver microsomes (HLM) at 1 mg/mL protein concentration, NADPH regeneration system (37°C, pH 7.4).
- Control for batch variability: Pre-screen HLMs for CYP450 activity.
- Quantify metabolites: LC-MS/MS with stable isotope-labeled internal standards.
- Statistical validation: Triplicate runs with coefficient of variation (CV) <15% .
Q. How to design a factorial experiment for structure-activity relationship (SAR) optimization?
Methodological Answer: Implement a 2 factorial design to evaluate critical substituents:
- Factors: Cyclohexyl ring conformation (chair vs. boat), tetrahydroquinoline N-substitution.
- Responses: Solubility (logP), metabolic half-life (t), and potency (IC).
- Analysis: ANOVA to identify interaction effects; Pareto charts to prioritize modifications. Use COMSOL Multiphysics for solubility-pH modeling .
Q. Theoretical and Methodological Frameworks
Q. How to align mechanistic studies with a conceptual framework for urea-based inhibitors?
Methodological Answer:
- Theoretical basis: Link to enzyme transition-state mimicry theory, where the urea group mimics ATP’s adenine-ribose motif in kinases.
- Experimental validation: Competitive inhibition assays with ATP gradients.
- Data interpretation: Use Lineweaver-Burk plots to distinguish competitive vs. non-competitive mechanisms .
Q. What methodologies reconcile conflicting cytotoxicity data across cell lines?
Methodological Answer:
- Hypothesis-driven stratification: Test cell lines with varying expression levels of putative targets (e.g., Western blot for kinase X).
- Microenvironment modulation: Compare 2D monolayers vs. 3D spheroids to assess penetration efficacy.
- Multi-omics integration: RNA-seq to identify resistance pathways (e.g., ABC transporter upregulation) .
Q. Data Analysis and Reporting
Q. How should researchers statistically validate outliers in pharmacokinetic (PK) studies?
Methodological Answer:
- Outlier detection: Grubbs’ test (α = 0.05) for AUC or C values.
- Causality analysis: Cross-check with formulation stability (e.g., particle size distribution) or animal health records.
- Reporting: Transparently document excluded data points in supplementary materials .
Q. What bibliometric approaches identify knowledge gaps in urea-based therapeutics?
Methodological Answer:
- Keyword clustering: Use VOSviewer to map co-occurrence terms (e.g., “urea derivative,” “kinase inhibition,” “resistance”).
- Citation analysis: Identify under-cited mechanistic studies for replication.
- Trend forecasting: Analyze publication growth rates (2010–2025) in Scopus/PubMed to prioritize underexplored targets (e.g., epigenetic regulators) .
Properties
IUPAC Name |
1-cyclohexyl-3-(1,2,3,4-tetrahydroquinolin-5-yl)urea | |
---|---|---|
Details | Computed by LexiChem 2.6.6 (PubChem release 2019.06.18) | |
Source | PubChem | |
URL | https://pubchem.ncbi.nlm.nih.gov | |
Description | Data deposited in or computed by PubChem | |
InChI |
InChI=1S/C16H23N3O/c20-16(18-12-6-2-1-3-7-12)19-15-10-4-9-14-13(15)8-5-11-17-14/h4,9-10,12,17H,1-3,5-8,11H2,(H2,18,19,20) | |
Details | Computed by InChI 1.0.5 (PubChem release 2019.06.18) | |
Source | PubChem | |
URL | https://pubchem.ncbi.nlm.nih.gov | |
Description | Data deposited in or computed by PubChem | |
InChI Key |
NDOJFQKSTXJQHE-UHFFFAOYSA-N | |
Details | Computed by InChI 1.0.5 (PubChem release 2019.06.18) | |
Source | PubChem | |
URL | https://pubchem.ncbi.nlm.nih.gov | |
Description | Data deposited in or computed by PubChem | |
Canonical SMILES |
C1CCC(CC1)NC(=O)NC2=CC=CC3=C2CCCN3 | |
Details | Computed by OEChem 2.1.5 (PubChem release 2019.06.18) | |
Source | PubChem | |
URL | https://pubchem.ncbi.nlm.nih.gov | |
Description | Data deposited in or computed by PubChem | |
Molecular Formula |
C16H23N3O | |
Details | Computed by PubChem 2.1 (PubChem release 2019.06.18) | |
Source | PubChem | |
URL | https://pubchem.ncbi.nlm.nih.gov | |
Description | Data deposited in or computed by PubChem | |
Molecular Weight |
273.37 g/mol | |
Details | Computed by PubChem 2.1 (PubChem release 2021.05.07) | |
Source | PubChem | |
URL | https://pubchem.ncbi.nlm.nih.gov | |
Description | Data deposited in or computed by PubChem | |
Disclaimer and Information on In-Vitro Research Products
Please be aware that all articles and product information presented on BenchChem are intended solely for informational purposes. The products available for purchase on BenchChem are specifically designed for in-vitro studies, which are conducted outside of living organisms. In-vitro studies, derived from the Latin term "in glass," involve experiments performed in controlled laboratory settings using cells or tissues. It is important to note that these products are not categorized as medicines or drugs, and they have not received approval from the FDA for the prevention, treatment, or cure of any medical condition, ailment, or disease. We must emphasize that any form of bodily introduction of these products into humans or animals is strictly prohibited by law. It is essential to adhere to these guidelines to ensure compliance with legal and ethical standards in research and experimentation.