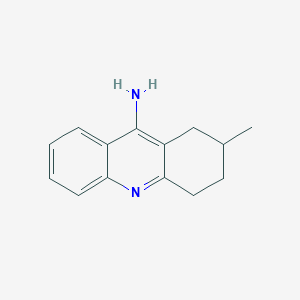
2-Methyl-1,2,3,4-tetrahydroacridin-9-amine
- Click on QUICK INQUIRY to receive a quote from our team of experts.
- With the quality product at a COMPETITIVE price, you can focus more on your research.
Overview
Description
Scientific Research Applications
Theoretical Study and Chemical Properties
- Theoretical Studies: 2-Methyl-1,2,3,4-tetrahydroacridin-9-amine (THA) has been studied theoretically for its structure, reactivity, and stability. Theoretical molecular orbital (MO) approaches have shown the stability and lack of reactivity of THA's amino group in both basic and protonated forms, which is attributed to electron delocalization. Such studies aid in understanding the chemical properties and potential pharmaceutical applications of THA (Pop, Brewster, Kaminski, & Bodor, 1989).
Synthesis and Chemical Reactions
- Synthesis Techniques: Advances in the synthesis of functionalized THA derivatives have been made, particularly using Buchwald–Hartwig amination. This method has been effective for synthesizing highly functionalized tacrines, demonstrating THA’s versatility in chemical synthesis (de Sousa, Brown, & Baati, 2014).
- Alkylation of C(sp3)–H and C(sp2)–H Bonds: THA derivatives have been used in palladium-catalyzed alkylation reactions. This application is significant in the preparation of various compounds, including unnatural amino acids and acrylic acids (Zhu, He, Wang, & Yu, 2014).
Biological and Pharmacological Applications
- Inhibition of Acetylcholinesterase: Some THA derivatives have shown potent and selective inhibition of acetylcholinesterase, a key target in Alzheimer’s disease therapy. These derivatives offer insights into the role of THA in treating Alzheimer’s disease and their potential as safer, cost-effective therapeutic options (Pang, Hong, Quiram, Jelacic, & Brimijoin, 1997).
- Blockage of Potassium Channels: Studies have demonstrated that THA can block cardiac potassium channels, affecting myocardial membrane currents. This highlights a potential pharmacological application of THA in cardiac therapies (Osterrieder, 1987).
Multifunctional Agents in Alzheimer’s Disease
- Structure-Activity Relationship Studies: A library of THA derivatives was synthesized and evaluated as dual inhibitors of cholinesterase and amyloid aggregation, crucial in Alzheimer's disease treatment. This research supports the development of THA-based multitargeting agents for Alzheimer’s disease (Osman, Mohamed, Sit, Vasefi, Beazely, & Rao, 2016).
Other Applications
- Antitumor Activity: Some THA derivatives have shown potential as antitumor agents, particularly in the field of DNA-intercalating agents used in cancer chemotherapy (Kumar, Sharma, Sharma, Silakari, Singh, & Kaur, 2017).
- Corrosion Inhibition: THA derivatives, combined with other compounds like Tween-80, have been investigated for their effectiveness in inhibiting mild steel corrosion in acidic mediums. This suggests industrial applications for THA in materials protection (Zhang, Wang, Li, Liu, Tao, Guan, Li, & Wu, 2019).
Mechanism of Action
Properties
IUPAC Name |
2-methyl-1,2,3,4-tetrahydroacridin-9-amine |
Source
|
---|---|---|
Details | Computed by LexiChem 2.6.6 (PubChem release 2019.06.18) | |
Source | PubChem | |
URL | https://pubchem.ncbi.nlm.nih.gov | |
Description | Data deposited in or computed by PubChem | |
InChI |
InChI=1S/C14H16N2/c1-9-6-7-13-11(8-9)14(15)10-4-2-3-5-12(10)16-13/h2-5,9H,6-8H2,1H3,(H2,15,16) |
Source
|
Details | Computed by InChI 1.0.5 (PubChem release 2019.06.18) | |
Source | PubChem | |
URL | https://pubchem.ncbi.nlm.nih.gov | |
Description | Data deposited in or computed by PubChem | |
InChI Key |
NCTPLHBPILFKIF-UHFFFAOYSA-N |
Source
|
Details | Computed by InChI 1.0.5 (PubChem release 2019.06.18) | |
Source | PubChem | |
URL | https://pubchem.ncbi.nlm.nih.gov | |
Description | Data deposited in or computed by PubChem | |
Canonical SMILES |
CC1CCC2=NC3=CC=CC=C3C(=C2C1)N |
Source
|
Details | Computed by OEChem 2.1.5 (PubChem release 2019.06.18) | |
Source | PubChem | |
URL | https://pubchem.ncbi.nlm.nih.gov | |
Description | Data deposited in or computed by PubChem | |
Molecular Formula |
C14H16N2 |
Source
|
Details | Computed by PubChem 2.1 (PubChem release 2019.06.18) | |
Source | PubChem | |
URL | https://pubchem.ncbi.nlm.nih.gov | |
Description | Data deposited in or computed by PubChem | |
Molecular Weight |
212.29 g/mol |
Source
|
Details | Computed by PubChem 2.1 (PubChem release 2021.05.07) | |
Source | PubChem | |
URL | https://pubchem.ncbi.nlm.nih.gov | |
Description | Data deposited in or computed by PubChem | |
Disclaimer and Information on In-Vitro Research Products
Please be aware that all articles and product information presented on BenchChem are intended solely for informational purposes. The products available for purchase on BenchChem are specifically designed for in-vitro studies, which are conducted outside of living organisms. In-vitro studies, derived from the Latin term "in glass," involve experiments performed in controlled laboratory settings using cells or tissues. It is important to note that these products are not categorized as medicines or drugs, and they have not received approval from the FDA for the prevention, treatment, or cure of any medical condition, ailment, or disease. We must emphasize that any form of bodily introduction of these products into humans or animals is strictly prohibited by law. It is essential to adhere to these guidelines to ensure compliance with legal and ethical standards in research and experimentation.