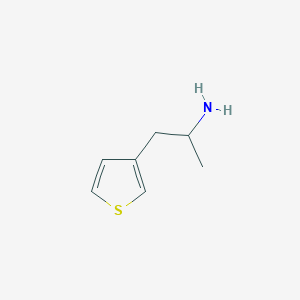
1-(Thiophen-3-yl)propan-2-amine
Overview
Description
1-(Thiophen-3-yl)propan-2-amine is a chemical compound that belongs to the class of thiophene derivatives. It is structurally similar to amphetamine, with the phenyl ring replaced by a thiophene ring.
Mechanism of Action
Target of Action
It is known that thiophene derivatives exhibit a variety of pharmacological properties such as anticancer, anti-inflammatory, antimicrobial, antihypertensive, and anti-atherosclerotic properties . Therefore, it can be inferred that 1-(Thiophen-3-yl)propan-2-amine may interact with a range of biological targets.
Mode of Action
It is suggested that like amphetamine and most of its analogues, thiopropamine most likely acts as a norepinephrine–dopamine reuptake inhibitor and/or releasing agent .
Biochemical Pathways
For instance, if it exhibits anti-inflammatory properties, it may interact with pathways involved in inflammation, such as the NF-kB pathway .
Pharmacokinetics
It is known that thiopropamine, a similar compound, is rapidly absorbed following intraperitoneal injection, with peak concentrations in the blood achieved at five minutes and a subsequent reduction to 12% of peak concentrations within two hours post-administration . It is also likely to be metabolized into active 4-hydroxymethiopropamine and thiophene S-oxides. These are further deaminated by CYP2C in the liver, transforming them into inactive 1-(Thiophen-2-yl)-2-propan-2-one, which is a phenylacetone derivative .
Biochemical Analysis
Biochemical Properties
1-(Thiophen-3-yl)propan-2-amine plays a significant role in biochemical reactions. It interacts with several enzymes, proteins, and other biomolecules. One of the key interactions is with the cytochrome P450 enzyme family, particularly CYP2C19, which is involved in the metabolism of various substances. The compound can act as a substrate for these enzymes, leading to its biotransformation into active metabolites such as thiopropamine and 4-hydroxymethiopropamine . These interactions are crucial for understanding the compound’s metabolic pathways and its potential therapeutic applications.
Metabolic Pathways
This compound is involved in several metabolic pathways. It is primarily metabolized by the cytochrome P450 enzyme family, particularly CYP2C19, leading to the formation of active metabolites such as thiopropamine and 4-hydroxymethiopropamine . These metabolites can further undergo biotransformation, resulting in the formation of inactive compounds such as 1-(thiophen-2-yl)-2-propan-2-one . The compound’s involvement in these metabolic pathways highlights its potential for therapeutic applications and its impact on metabolic health.
Preparation Methods
Synthetic Routes and Reaction Conditions: The synthesis of 1-(Thiophen-3-yl)propan-2-amine typically involves the following steps:
Starting Material: The synthesis begins with thiophene, a five-membered aromatic ring containing one sulfur atom.
Alkylation: Thiophene undergoes alkylation to introduce a propylamine side chain at the 3-position. This can be achieved using reagents such as propylamine and a suitable catalyst.
Purification: The final product is purified using techniques like recrystallization or chromatography to obtain pure this compound.
Industrial Production Methods: Industrial production of this compound may involve large-scale alkylation reactions using continuous flow reactors to ensure consistent quality and yield. The use of automated systems and advanced purification techniques can enhance the efficiency of the production process .
Chemical Reactions Analysis
Types of Reactions: 1-(Thiophen-3-yl)propan-2-amine undergoes various chemical reactions, including:
Oxidation: The compound can be oxidized to form sulfoxides or sulfones using oxidizing agents like hydrogen peroxide or m-chloroperbenzoic acid.
Reduction: Reduction reactions can convert the compound into its corresponding thiol or thioether derivatives using reducing agents such as lithium aluminum hydride.
Common Reagents and Conditions:
Oxidation: Hydrogen peroxide, m-chloroperbenzoic acid.
Reduction: Lithium aluminum hydride, sodium borohydride.
Substitution: Alkyl halides, acyl chlorides.
Major Products Formed:
Oxidation: Sulfoxides, sulfones.
Reduction: Thiols, thioethers.
Substitution: N-alkylated or N-acylated derivatives.
Scientific Research Applications
1-(Thiophen-3-yl)propan-2-amine has several scientific research applications:
Comparison with Similar Compounds
- Thiopropamine
- Methiopropamine
Biological Activity
1-(Thiophen-3-yl)propan-2-amine, often referred to in the context of its analogs such as methiopropamine (N-methyl-1-(thiophen-2-yl)propan-2-amine), has garnered attention due to its psychoactive properties and potential implications for public health. This article explores the biological activity of this compound, focusing on its pharmacological effects, toxicity, and case studies reflecting its impact on human health.
This compound is structurally related to amphetamines, characterized by a thiophene ring. The compound's structure suggests potential interactions with neurotransmitter systems, particularly those involving monoamines.
Pharmacological Activity
The biological activity of this compound can be examined through its influence on various receptors and neurotransmitter systems:
- Dopaminergic Activity : Similar compounds have been shown to act as dopamine reuptake inhibitors, potentially leading to increased levels of dopamine in the synaptic cleft, which may contribute to stimulant effects.
- Serotonergic Activity : Some studies indicate that related compounds can also affect serotonin receptors, which may lead to altered mood and perception.
Toxicological Findings
The toxicity profile of this compound has been assessed through various case studies, particularly focusing on instances of misuse. The following table summarizes key findings from reported cases:
Case Study | Symptoms | Plasma Concentration | Outcome |
---|---|---|---|
Case 1 (2012) | Palpitations, anxiety, hallucinations | 400 ng/mL (methiopropamine) | Discharged after treatment |
Case 2 (2014) | Chest pain, paranoia, visual hallucinations | Not specified; multiple substances detected | Hospitalized but stabilized |
Case 3 (2016) | Nausea, vomiting, agitation | 3.7 mg/L (methiopropamine) | Fatal; other drugs involved |
Clinical Implications
The clinical implications of using compounds like this compound are significant. Reports indicate that users may experience a range of adverse effects including:
- Cardiovascular Issues : Increased heart rate and blood pressure have been noted in users.
- Psychological Effects : Anxiety, paranoia, and hallucinations are common among users, particularly at higher doses.
Regulatory Status
Due to its psychoactive properties and associated risks, regulatory bodies have taken steps to control substances like methiopropamine. In the UK, it has been classified under the Misuse of Drugs Act due to concerns over its safety profile and potential for abuse .
Properties
IUPAC Name |
1-thiophen-3-ylpropan-2-amine | |
---|---|---|
Details | Computed by LexiChem 2.6.6 (PubChem release 2019.06.18) | |
Source | PubChem | |
URL | https://pubchem.ncbi.nlm.nih.gov | |
Description | Data deposited in or computed by PubChem | |
InChI |
InChI=1S/C7H11NS/c1-6(8)4-7-2-3-9-5-7/h2-3,5-6H,4,8H2,1H3 | |
Details | Computed by InChI 1.0.5 (PubChem release 2019.06.18) | |
Source | PubChem | |
URL | https://pubchem.ncbi.nlm.nih.gov | |
Description | Data deposited in or computed by PubChem | |
InChI Key |
RTEQDZAVAQTCOK-UHFFFAOYSA-N | |
Details | Computed by InChI 1.0.5 (PubChem release 2019.06.18) | |
Source | PubChem | |
URL | https://pubchem.ncbi.nlm.nih.gov | |
Description | Data deposited in or computed by PubChem | |
Canonical SMILES |
CC(CC1=CSC=C1)N | |
Details | Computed by OEChem 2.1.5 (PubChem release 2019.06.18) | |
Source | PubChem | |
URL | https://pubchem.ncbi.nlm.nih.gov | |
Description | Data deposited in or computed by PubChem | |
Molecular Formula |
C7H11NS | |
Details | Computed by PubChem 2.1 (PubChem release 2019.06.18) | |
Source | PubChem | |
URL | https://pubchem.ncbi.nlm.nih.gov | |
Description | Data deposited in or computed by PubChem | |
DSSTOX Substance ID |
DTXSID401344776 | |
Record name | 3-Thienoamphetamine | |
Source | EPA DSSTox | |
URL | https://comptox.epa.gov/dashboard/DTXSID401344776 | |
Description | DSSTox provides a high quality public chemistry resource for supporting improved predictive toxicology. | |
Molecular Weight |
141.24 g/mol | |
Details | Computed by PubChem 2.1 (PubChem release 2021.05.07) | |
Source | PubChem | |
URL | https://pubchem.ncbi.nlm.nih.gov | |
Description | Data deposited in or computed by PubChem | |
CAS No. |
149977-81-1 | |
Record name | 3-Thienoamphetamine | |
Source | EPA DSSTox | |
URL | https://comptox.epa.gov/dashboard/DTXSID401344776 | |
Description | DSSTox provides a high quality public chemistry resource for supporting improved predictive toxicology. | |
Record name | 1-(thiophen-3-yl)propan-2-amine | |
Source | European Chemicals Agency (ECHA) | |
URL | https://echa.europa.eu/information-on-chemicals | |
Description | The European Chemicals Agency (ECHA) is an agency of the European Union which is the driving force among regulatory authorities in implementing the EU's groundbreaking chemicals legislation for the benefit of human health and the environment as well as for innovation and competitiveness. | |
Explanation | Use of the information, documents and data from the ECHA website is subject to the terms and conditions of this Legal Notice, and subject to other binding limitations provided for under applicable law, the information, documents and data made available on the ECHA website may be reproduced, distributed and/or used, totally or in part, for non-commercial purposes provided that ECHA is acknowledged as the source: "Source: European Chemicals Agency, http://echa.europa.eu/". Such acknowledgement must be included in each copy of the material. ECHA permits and encourages organisations and individuals to create links to the ECHA website under the following cumulative conditions: Links can only be made to webpages that provide a link to the Legal Notice page. | |
Disclaimer and Information on In-Vitro Research Products
Please be aware that all articles and product information presented on BenchChem are intended solely for informational purposes. The products available for purchase on BenchChem are specifically designed for in-vitro studies, which are conducted outside of living organisms. In-vitro studies, derived from the Latin term "in glass," involve experiments performed in controlled laboratory settings using cells or tissues. It is important to note that these products are not categorized as medicines or drugs, and they have not received approval from the FDA for the prevention, treatment, or cure of any medical condition, ailment, or disease. We must emphasize that any form of bodily introduction of these products into humans or animals is strictly prohibited by law. It is essential to adhere to these guidelines to ensure compliance with legal and ethical standards in research and experimentation.