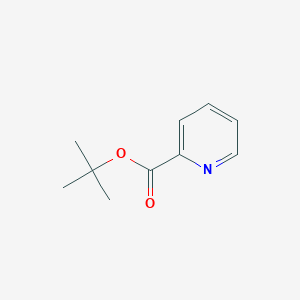
Tert-butyl Pyridine-2-carboxylate
Overview
Description
Tert-butyl pyridine-2-carboxylate is a pyridine derivative characterized by a tert-butyl ester group attached to the carboxylate moiety at the 2-position of the pyridine ring. Its molecular formula is C₁₃H₂₄N₂O₂, comprising 41 atoms (13 carbons, 24 hydrogens, 2 nitrogens, and 2 oxygens) . The compound exhibits stereochemical complexity, as demonstrated by its bicyclic structure with a fused cyclopenta[c]pyridine system and an octahydro backbone in specific stereoisomeric forms (e.g., (4aR,6R,7aS)) . This structure grants unique steric and electronic properties, making it a versatile intermediate in organic synthesis and pharmaceutical applications.
Preparation Methods
Synthetic Routes and Reaction Conditions
Tert-butyl Pyridine-2-carboxylate can be synthesized through several methods. One common approach involves the reaction of pyridine-2-carboxylic acid with tert-butyl alcohol in the presence of a dehydrating agent such as thionyl chloride or phosphorus trichloride. The reaction typically occurs under reflux conditions, and the product is purified through distillation or recrystallization.
Industrial Production Methods
In an industrial setting, the production of this compound may involve continuous flow processes to enhance efficiency and yield. The use of automated reactors and precise control of reaction parameters can lead to higher purity and consistency in the final product.
Chemical Reactions Analysis
Types of Reactions
Tert-butyl Pyridine-2-carboxylate undergoes various chemical reactions, including:
Oxidation: The compound can be oxidized to form pyridine-2-carboxylic acid derivatives.
Reduction: Reduction reactions can convert the carboxylate group to an alcohol or aldehyde.
Substitution: The tert-butyl group can be substituted with other functional groups through nucleophilic substitution reactions.
Common Reagents and Conditions
Oxidation: Common oxidizing agents include potassium permanganate and chromium trioxide.
Reduction: Reducing agents such as lithium aluminum hydride or sodium borohydride are often used.
Substitution: Nucleophiles like amines or thiols can be employed under basic conditions to achieve substitution.
Major Products
The major products formed from these reactions depend on the specific reagents and conditions used. For example, oxidation may yield pyridine-2-carboxylic acid, while reduction can produce pyridine-2-methanol.
Scientific Research Applications
Medicinal Chemistry
Pharmaceutical Development
Tert-butyl pyridine-2-carboxylate serves as a lead compound in the development of new pharmaceuticals. Its structural properties allow it to interact with biological targets effectively, making it a candidate for drugs aimed at treating various diseases, including pain management and inflammation.
- Case Study: TRPV1 Antagonism
Research has shown that derivatives of tert-butyl pyridine exhibit antagonistic activity against the transient receptor potential vanilloid 1 (TRPV1), which is implicated in pain pathways. For instance, specific analogs demonstrated a potent inhibition of capsaicin-induced activation, with one compound showing an value of 0.1 nM, indicating strong binding affinity and potential for analgesic applications .
Agricultural Chemistry
Pesticide Development
The compound has been explored for use in developing agrochemicals, particularly as a pesticide or herbicide. Its efficacy in inhibiting certain biological processes in pests suggests it could be engineered into effective agricultural products.
- Research Insights
Studies indicate that modifications to the tert-butyl pyridine structure can enhance its bioactivity against specific pests while minimizing environmental impact. This adaptability makes it suitable for sustainable agricultural practices.
Materials Science
Synthesis of Advanced Materials
this compound is utilized as a building block in synthesizing more complex organic molecules and materials. Its ability to form coordination complexes enhances the development of novel materials with tailored properties.
- Example Application: Coordination Chemistry
The compound can act as a ligand in coordination chemistry, forming complexes with transition metals that exhibit unique electronic and optical properties. These complexes have potential applications in catalysis and sensor technology.
Data Table: Comparison of Biological Activities
Compound Name | Activity Type | (nM) | Reference |
---|---|---|---|
This compound | TRPV1 Antagonist | 0.1 | |
Derivative A | TRPV1 Antagonist | 0.7 | |
Derivative B | Herbicidal Activity | - | Emerging Research |
Synthesis and Characterization
The synthesis of this compound typically involves multi-step reactions that can be optimized for yield and purity. Techniques such as chromatography and NMR spectroscopy are employed to characterize the final product.
Mechanism of Action
The mechanism by which Tert-butyl Pyridine-2-carboxylate exerts its effects involves its interaction with molecular targets such as enzymes or receptors. The tert-butyl group can enhance the compound’s lipophilicity, allowing it to penetrate biological membranes more effectively. The pyridine ring can participate in hydrogen bonding and π-π interactions, stabilizing the compound’s binding to its target.
Comparison with Similar Compounds
Comparison with Similar Pyridine Carboxylates
Structural Variations and Substituent Effects
Tert-butyl pyridine-2-carboxylate belongs to a broader class of pyridine carboxylate esters. Key structural analogs include:
Key Observations :
- Electron-Withdrawing Groups : Chlorine or nitro substituents (e.g., in bromo-nitrobenzene derivatives) enhance reactivity in Pd-catalyzed coupling reactions, as seen in .
- Coordination Chemistry : Pyridine-2-carboxylate ligands form stable complexes with metals like Sn, Ge, and Si, with ¹¹⁹Sn NMR shifts indicative of heptacoordination (−617 to −620 ppm) .
Biological Activity
Tert-butyl pyridine-2-carboxylate (TBPC) is a heterocyclic compound with significant potential in medicinal chemistry. Its molecular formula is CHNO, and it has garnered attention for its diverse biological activities. This article explores the biological activity of TBPC, including its pharmacological properties, mechanisms of action, and relevant case studies.
Chemical Structure and Properties
TBPC features a pyridine ring substituted with a tert-butyl group and a carboxylate moiety. The structural characteristics contribute to its chemical reactivity and biological interactions. The compound's molecular weight is approximately 179.22 g/mol, which influences its pharmacokinetics and bioavailability.
Biological Activity Overview
Research indicates that TBPC exhibits a range of biological activities, including:
- Antimicrobial Activity : Preliminary studies suggest that TBPC may possess antimicrobial properties against various pathogens.
- Anticancer Potential : Some derivatives of pyridine compounds have shown promise in inhibiting cancer cell proliferation.
- Anti-inflammatory Effects : TBPC may modulate inflammatory pathways, although specific mechanisms remain to be elucidated.
The biological activity of TBPC can be attributed to several mechanisms:
- Enzyme Inhibition : TBPC may act as an inhibitor of specific enzymes involved in metabolic processes or pathogen survival.
- Receptor Modulation : The compound could interact with various receptors, influencing cellular signaling pathways.
- Oxidative Stress Reduction : Some studies suggest that TBPC might exhibit antioxidant properties, mitigating oxidative damage in cells.
Antimicrobial Activity
A study investigated the antimicrobial efficacy of TBPC against common bacterial strains. Results indicated that TBPC demonstrated significant inhibitory effects on Gram-positive bacteria, particularly Staphylococcus aureus, with a minimum inhibitory concentration (MIC) of 32 µg/mL. The study highlighted the potential for TBPC as a lead compound for developing new antibiotics.
Bacterial Strain | Minimum Inhibitory Concentration (µg/mL) |
---|---|
Staphylococcus aureus | 32 |
Escherichia coli | 64 |
Pseudomonas aeruginosa | 128 |
Anticancer Activity
In another investigation, TBPC derivatives were tested for their ability to inhibit cancer cell lines. The results showed that certain modifications to the pyridine structure enhanced cytotoxicity against breast cancer cells (MCF-7), with IC values ranging from 10 to 20 µM.
Compound | IC (µM) | Cancer Cell Line |
---|---|---|
TBPC | 15 | MCF-7 |
Modified Derivative A | 10 | MCF-7 |
Modified Derivative B | 20 | MCF-7 |
Q & A
Basic Research Questions
Q. What are the optimal synthetic routes for tert-butyl pyridine-2-carboxylate derivatives?
- Methodological Answer: Common methods include esterification, nucleophilic substitution, and cross-coupling. For example, ester hydrolysis under acidic (HCl/THF/H₂O) or basic (NaOH) conditions can yield carboxylic acids. Cross-coupling with Pd(PPh₃)₄ and aryl halides produces arylated derivatives . Purification often involves chromatography or crystallization .
Q. How do reaction conditions influence the hydrolysis of this compound esters?
- Methodological Answer: Hydrolysis efficiency depends on steric hindrance and electronic effects. Acidic conditions (HCl) are preferred for bulky esters, while basic conditions (NaOH) suit less hindered derivatives. Reaction monitoring via TLC or NMR is critical to optimize time and temperature .
Q. What spectroscopic techniques are essential for characterizing this compound derivatives?
- Methodological Answer:
- NMR: ¹H/¹³C NMR identifies substitution patterns and confirms tert-butyl group integrity (δ ~1.2 ppm for tert-butyl protons).
- IR: Confirms carbonyl stretches (~1700 cm⁻¹ for esters).
- MS: High-resolution mass spectrometry validates molecular formulas .
Q. How can researchers purify this compound derivatives effectively?
- Methodological Answer: Column chromatography (silica gel, hexane/EtOAc gradient) isolates products. For crystalline derivatives, recrystallization in ethanol or acetone improves purity .
Advanced Research Questions
Q. How can contradictory data on reaction yields for this compound syntheses be resolved?
- Methodological Answer: Systematically vary parameters (catalyst loading, temperature, solvent) in controlled experiments. For example, cross-coupling yields may improve with Cs₂CO₃ as a base versus K₂CO₃ due to enhanced solubility . Statistical tools like Design of Experiments (DoE) can identify critical factors.
Q. What strategies mitigate competing side reactions during nucleophilic substitution?
- Methodological Answer: Use low temperatures (0°C) and strong bases (NaH) to minimize elimination. Pre-activation of electrophiles (e.g., alkyl bromides) and anhydrous conditions reduce hydrolysis byproducts .
Q. How do electronic effects of substituents impact the reactivity of this compound in cross-coupling?
- Methodological Answer: Electron-withdrawing groups (e.g., -Cl) enhance electrophilicity at the pyridine ring, improving coupling efficiency. Hammett plots or computational DFT studies can quantify substituent effects .
Q. What mechanistic insights explain the regioselectivity of this compound oxidation?
- Methodological Answer: Oxidation with Dess–Martin periodinane targets α-C positions adjacent to the ester group. Steric shielding by the tert-butyl group directs oxidation to less hindered sites. Kinetic studies and isotopic labeling (e.g., ¹⁸O) validate pathways .
Application-Oriented Questions
Q. How can this compound derivatives be tailored for kinase inhibition studies?
- Methodological Answer: Introduce bioisosteres (e.g., fluorine) at the pyridine ring to enhance binding. Molecular docking with kinase ATP pockets guides structural modifications. In vitro assays (e.g., ADP-Glo™) quantify inhibitory activity .
Q. What comparative analyses distinguish this compound analogs in enzyme inhibition?
- Methodological Answer: Compare IC₅₀ values against structurally similar compounds (e.g., dichloropyridinyl carbamates). QSAR models correlate substituent electronic parameters (σ, π) with activity .
Q. Data Analysis and Optimization
Q. How to design a high-throughput screening protocol for this compound derivatives?
- Methodological Answer: Use automated liquid handlers for parallel synthesis. LC-MS/MS monitors reaction progress. Machine learning algorithms (e.g., Random Forest) predict optimal conditions from historical data .
Q. What computational tools aid in predicting the stability of this compound derivatives?
- Methodological Answer: Molecular dynamics (MD) simulations assess conformational stability. DFT calculations (e.g., B3LYP/6-31G*) evaluate hydrolysis activation energies .
Q. Table: Key Reaction Conditions for Functionalization
Properties
IUPAC Name |
tert-butyl pyridine-2-carboxylate | |
---|---|---|
Details | Computed by LexiChem 2.6.6 (PubChem release 2019.06.18) | |
Source | PubChem | |
URL | https://pubchem.ncbi.nlm.nih.gov | |
Description | Data deposited in or computed by PubChem | |
InChI |
InChI=1S/C10H13NO2/c1-10(2,3)13-9(12)8-6-4-5-7-11-8/h4-7H,1-3H3 | |
Details | Computed by InChI 1.0.5 (PubChem release 2019.06.18) | |
Source | PubChem | |
URL | https://pubchem.ncbi.nlm.nih.gov | |
Description | Data deposited in or computed by PubChem | |
InChI Key |
KNLKRAUJQBLECR-UHFFFAOYSA-N | |
Details | Computed by InChI 1.0.5 (PubChem release 2019.06.18) | |
Source | PubChem | |
URL | https://pubchem.ncbi.nlm.nih.gov | |
Description | Data deposited in or computed by PubChem | |
Canonical SMILES |
CC(C)(C)OC(=O)C1=CC=CC=N1 | |
Details | Computed by OEChem 2.1.5 (PubChem release 2019.06.18) | |
Source | PubChem | |
URL | https://pubchem.ncbi.nlm.nih.gov | |
Description | Data deposited in or computed by PubChem | |
Molecular Formula |
C10H13NO2 | |
Details | Computed by PubChem 2.1 (PubChem release 2019.06.18) | |
Source | PubChem | |
URL | https://pubchem.ncbi.nlm.nih.gov | |
Description | Data deposited in or computed by PubChem | |
Molecular Weight |
179.22 g/mol | |
Details | Computed by PubChem 2.1 (PubChem release 2021.05.07) | |
Source | PubChem | |
URL | https://pubchem.ncbi.nlm.nih.gov | |
Description | Data deposited in or computed by PubChem | |
Disclaimer and Information on In-Vitro Research Products
Please be aware that all articles and product information presented on BenchChem are intended solely for informational purposes. The products available for purchase on BenchChem are specifically designed for in-vitro studies, which are conducted outside of living organisms. In-vitro studies, derived from the Latin term "in glass," involve experiments performed in controlled laboratory settings using cells or tissues. It is important to note that these products are not categorized as medicines or drugs, and they have not received approval from the FDA for the prevention, treatment, or cure of any medical condition, ailment, or disease. We must emphasize that any form of bodily introduction of these products into humans or animals is strictly prohibited by law. It is essential to adhere to these guidelines to ensure compliance with legal and ethical standards in research and experimentation.