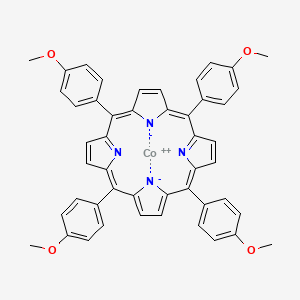
Cobalt tetramethoxyphenylporphyrin
- Click on QUICK INQUIRY to receive a quote from our team of experts.
- With the quality product at a COMPETITIVE price, you can focus more on your research.
Overview
Description
Cobalt tetramethoxyphenylporphyrin is an organometallic compound with the chemical formula C48H36CoN4O4 . It is a derivative of porphyrin, a macrocyclic compound that plays a crucial role in various biological systems. This compound is known for its deep red or purple color and its ability to dissolve in common organic solvents like chloroform and dichloromethane . This compound is widely used in chemical research, particularly as a catalyst in organic synthesis reactions .
Mechanism of Action
Target of Action
The primary targets of Cobalt Tetramethoxyphenylporphyrin are protons and water molecules . It is used as a catalyst in electrochemical reactions, specifically for the reduction of protons or water .
Mode of Action
This compound interacts with its targets (protons or water molecules) through an electrochemical process . It facilitates the reduction of these targets, leading to the production of hydrogen .
Biochemical Pathways
The main biochemical pathway affected by this compound is the hydrogen evolution pathway . By catalyzing the reduction of protons or water, it promotes the production of hydrogen, a clean and renewable energy source .
Result of Action
The molecular effect of this compound’s action is the production of hydrogen through the reduction of protons or water
Action Environment
The efficacy and stability of this compound can be influenced by environmental factors such as the pH of the solution it is in . For instance, it has been shown to exhibit better activity in neutral buffered aqueous solution (pH 7.0) compared to an acetic acid solution .
Biochemical Analysis
Biochemical Properties
Cobalt tetramethoxyphenylporphyrin plays a significant role in biochemical reactions, particularly as a catalyst in oxidation processes. It interacts with various enzymes, proteins, and other biomolecules, facilitating the transfer of electrons and promoting redox reactions. One of the key interactions of this compound is with nitric oxide synthase, where it acts as a catalyst in the binding and activation of nitric oxide . Additionally, this compound has been shown to interact with cytochrome P450 enzymes, enhancing their catalytic activity in the oxidation of organic substrates .
Cellular Effects
This compound influences various cellular processes, including cell signaling pathways, gene expression, and cellular metabolism. It has been observed to affect the expression of genes involved in oxidative stress response and cellular defense mechanisms . In particular, this compound can modulate the activity of transcription factors such as nuclear factor erythroid 2-related factor 2 (Nrf2), leading to increased expression of antioxidant enzymes . This compound also impacts cellular metabolism by altering the activity of key metabolic enzymes, thereby influencing energy production and utilization within cells .
Molecular Mechanism
The molecular mechanism of action of this compound involves its ability to bind to specific biomolecules and modulate their activity. It acts as an electron donor or acceptor, facilitating redox reactions and influencing the activity of enzymes and other proteins. For example, this compound can inhibit the activity of certain enzymes by binding to their active sites and blocking substrate access . Additionally, it can activate enzymes by stabilizing their active conformations and promoting catalytic activity . These interactions result in changes in gene expression and cellular function, contributing to the overall biochemical effects of this compound.
Temporal Effects in Laboratory Settings
In laboratory settings, the effects of this compound can change over time due to its stability and degradation properties. Studies have shown that this compound is relatively stable under standard laboratory conditions, with minimal degradation observed over extended periods . Its long-term effects on cellular function can vary depending on the specific experimental conditions and the duration of exposure. In vitro studies have demonstrated that prolonged exposure to this compound can lead to changes in cellular metabolism and gene expression, potentially resulting in altered cellular function .
Dosage Effects in Animal Models
The effects of this compound in animal models vary with different dosages. At low doses, this compound has been shown to enhance the activity of antioxidant enzymes and improve cellular defense mechanisms . At high doses, it can exhibit toxic effects, including oxidative stress and cellular damage . Threshold effects have been observed, where the beneficial effects of this compound are seen at lower doses, while adverse effects become prominent at higher doses .
Metabolic Pathways
This compound is involved in various metabolic pathways, including those related to oxidative stress response and energy metabolism. It interacts with enzymes such as cytochrome P450 and nitric oxide synthase, influencing their activity and modulating metabolic flux . Additionally, this compound can affect the levels of metabolites involved in redox reactions, contributing to its overall biochemical effects .
Transport and Distribution
Within cells and tissues, this compound is transported and distributed through interactions with specific transporters and binding proteins. It can bind to albumin and other plasma proteins, facilitating its transport in the bloodstream . Additionally, this compound can interact with cellular transporters, such as organic anion-transporting polypeptides (OATPs), which mediate its uptake into cells . These interactions influence the localization and accumulation of this compound within different cellular compartments and tissues.
Subcellular Localization
The subcellular localization of this compound is influenced by its interactions with specific targeting signals and post-translational modifications. It has been observed to localize in the mitochondria, where it can influence mitochondrial function and energy production . Additionally, this compound can be found in the cytoplasm and nucleus, where it interacts with various biomolecules and modulates cellular processes. The specific localization of this compound within cells is critical for its biochemical activity and overall effects on cellular function.
Preparation Methods
Synthetic Routes and Reaction Conditions
Cobalt tetramethoxyphenylporphyrin can be synthesized by reacting 5,10,15,20-tetrakis(4-methoxyphenyl)porphyrin with cobalt acetate in glacial acetic acid . The reaction typically involves boiling the mixture to facilitate the formation of the cobalt complex. The resulting product is then purified through recrystallization or chromatography to obtain the desired compound in high purity .
Industrial Production Methods
While specific industrial production methods for this compound are not widely documented, the general approach involves scaling up the laboratory synthesis process. This includes optimizing reaction conditions, such as temperature, pressure, and solvent choice, to ensure efficient and cost-effective production. Industrial methods may also incorporate continuous flow reactors and automated purification systems to enhance yield and purity.
Chemical Reactions Analysis
Types of Reactions
Cobalt tetramethoxyphenylporphyrin undergoes various chemical reactions, including:
Oxidation: It can act as an oxidation catalyst in the selective oxidation of amines.
Reduction: It is used in the reduction of protons or water to form hydrogen.
Substitution: The compound can participate in substitution reactions, where ligands on the cobalt center are replaced by other groups.
Common Reagents and Conditions
Common reagents used in reactions with this compound include:
Cobalt acetate: Used in the synthesis of the compound.
Acetic acid: Serves as a solvent and reactant in the preparation process.
Hydrogen: Involved in reduction reactions to produce hydrogen gas.
Major Products Formed
The major products formed from reactions involving this compound depend on the specific reaction conditions. For example, in oxidation reactions, the primary products are oxidized amines, while in reduction reactions, hydrogen gas is produced .
Scientific Research Applications
Cobalt tetramethoxyphenylporphyrin has a wide range of applications in scientific research:
Comparison with Similar Compounds
Cobalt tetramethoxyphenylporphyrin can be compared with other similar compounds, such as:
Cobalt tetraphenylporphyrin: Similar in structure but lacks the methoxy groups, which can affect its solubility and reactivity.
Cobalt tetra(4-chlorophenyl)porphyrin: Contains chlorine substituents instead of methoxy groups, leading to different electronic properties and catalytic activities.
Cobalt tetra(4-carboxyphenyl)porphyrin: Features carboxyl groups that enhance its water solubility and make it suitable for aqueous-phase reactions.
This compound is unique due to its methoxy substituents, which influence its solubility, electronic properties, and catalytic behavior. These characteristics make it a versatile compound for various applications in chemistry, biology, medicine, and industry.
Biological Activity
Cobalt tetramethoxyphenylporphyrin (CoTMPP) is a cobalt(II) porphyrin complex that has garnered attention for its diverse biological activities and applications in various fields, including catalysis, medicine, and environmental science. This article explores the biological activity of CoTMPP, focusing on its mechanisms of action, cellular effects, and potential therapeutic applications.
1. Overview of this compound
CoTMPP is synthesized through a multi-step process involving cobalt acetate and 4-methoxybenzaldehyde, among other reagents. It is characterized by its purple color and is soluble in organic solvents. The compound has been investigated for its catalytic properties as well as its role in biological systems.
Target Interactions
CoTMPP primarily interacts with protons and water molecules through electrochemical processes. Its ability to bind to specific biomolecules allows it to modulate various biochemical pathways, particularly those related to electron transfer and redox reactions .
Biochemical Pathways
The main biochemical pathway influenced by CoTMPP is the hydrogen evolution pathway. This compound facilitates the production of hydrogen by reducing protons or water, which is critical for applications in energy conversion technologies.
3. Cellular Effects
CoTMPP has shown significant effects on cellular processes:
- Gene Expression: Research indicates that CoTMPP influences the expression of genes involved in oxidative stress responses and cellular defense mechanisms. This suggests a protective role against oxidative damage.
- Cell Signaling: The compound has been observed to affect various cell signaling pathways, potentially impacting cellular metabolism and growth.
4. Applications in Medicine
Therapeutic Potential
Ongoing studies are exploring the use of CoTMPP as a therapeutic agent. Its ability to mimic biological processes such as oxygen transport positions it as a candidate for drug development targeting specific biological pathways . Additionally, CoTMPP's role in nitric oxide synthase activation highlights its potential in cardiovascular therapies .
5. Catalytic Properties
CoTMPP serves as an efficient catalyst in several chemical reactions:
- Electrochemical Reactions: It has been utilized in the electrochemical reduction of carbon dioxide into useful products, demonstrating high selectivity and stability .
- Oxidation Catalysis: The compound is effective in catalyzing oxidation reactions, making it valuable in synthetic chemistry .
Case Study 1: Electrochemical CO2 Reduction
A study demonstrated that immobilized CoTMPP exhibits high catalytic activity for the electrochemical reduction of CO2 to carbon monoxide (CO), achieving a turnover frequency (TOF) of approximately 1.5 s−1 under optimized conditions. This study emphasizes the impact of peripheral substituents on catalytic efficiency .
Case Study 2: Gene Regulation
In cellular models, CoTMPP was shown to upregulate genes associated with oxidative stress response, suggesting its potential as a protective agent against oxidative injury in various diseases.
7. Safety and Hazards
While CoTMPP shows promising biological activities, it is essential to consider safety aspects. Studies have indicated that cobalt complexes can exhibit toxicity at high concentrations; thus, careful evaluation of dosage and exposure is necessary for therapeutic applications.
8. Future Directions
Research into CoTMPP continues to expand, focusing on:
- Enhanced Catalytic Systems: Developing hybrid systems combining CoTMPP with nanomaterials to improve catalytic performance.
- Therapeutic Applications: Investigating the use of CoTMPP in treating diseases related to oxidative stress and cardiovascular conditions.
Properties
CAS No. |
28903-71-1 |
---|---|
Molecular Formula |
C48H36CoN4O4 |
Molecular Weight |
791.8 g/mol |
IUPAC Name |
cobalt(2+);5,10,15,20-tetrakis(4-methoxyphenyl)porphyrin-22,24-diide |
InChI |
InChI=1S/C48H36N4O4.Co/c1-53-33-13-5-29(6-14-33)45-37-21-23-39(49-37)46(30-7-15-34(54-2)16-8-30)41-25-27-43(51-41)48(32-11-19-36(56-4)20-12-32)44-28-26-42(52-44)47(40-24-22-38(45)50-40)31-9-17-35(55-3)18-10-31;/h5-28H,1-4H3;/q-2;+2 |
InChI Key |
QBCIMRXPMLWVML-UHFFFAOYSA-N |
SMILES |
COC1=CC=C(C=C1)C2=C3C=CC(=C(C4=NC(=C(C5=CC=C([N-]5)C(=C6C=CC2=N6)C7=CC=C(C=C7)OC)C8=CC=C(C=C8)OC)C=C4)C9=CC=C(C=C9)OC)[N-]3.[Co+2] |
Canonical SMILES |
COC1=CC=C(C=C1)C2=C3C=CC(=C(C4=NC(=C(C5=CC=C([N-]5)C(=C6C=CC2=N6)C7=CC=C(C=C7)OC)C8=CC=C(C=C8)OC)C=C4)C9=CC=C(C=C9)OC)[N-]3.[Co+2] |
solubility |
not available |
Origin of Product |
United States |
Disclaimer and Information on In-Vitro Research Products
Please be aware that all articles and product information presented on BenchChem are intended solely for informational purposes. The products available for purchase on BenchChem are specifically designed for in-vitro studies, which are conducted outside of living organisms. In-vitro studies, derived from the Latin term "in glass," involve experiments performed in controlled laboratory settings using cells or tissues. It is important to note that these products are not categorized as medicines or drugs, and they have not received approval from the FDA for the prevention, treatment, or cure of any medical condition, ailment, or disease. We must emphasize that any form of bodily introduction of these products into humans or animals is strictly prohibited by law. It is essential to adhere to these guidelines to ensure compliance with legal and ethical standards in research and experimentation.