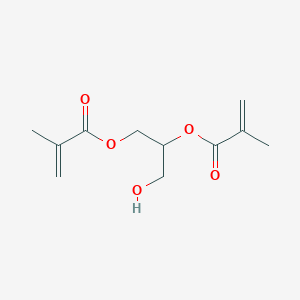
Glycerol 1,2-dimethacrylate
Overview
Description
Glycerol 1,2-dimethacrylate (GDMA), also known as glyceryl dimethacrylate or 2-hydroxy-1,3-propanediyl bismethacrylate, is a bifunctional methacrylate monomer with the CAS number 1830-78-0 . Structurally, it consists of a glycerol backbone substituted with two methacrylate groups, typically existing as a mixture of 1,2- and 1,3-isomers stabilized with MEHQ (4-methoxyphenol) to prevent premature polymerization .
Preparation Methods
Synthetic Routes and Reaction Conditions: Glycerol 1,2-dimethacrylate can be synthesized through the esterification of glycerol with methacrylic acid. The reaction typically involves the use of a catalyst, such as sulfuric acid or p-toluenesulfonic acid, to facilitate the esterification process. The reaction is carried out under reflux conditions, with the removal of water to drive the reaction to completion. The product is then purified through distillation or recrystallization .
Industrial Production Methods: In industrial settings, this compound is produced using similar esterification processes but on a larger scale. Continuous reactors and efficient separation techniques are employed to ensure high yield and purity of the product. The use of advanced catalysts and optimized reaction conditions further enhances the efficiency of the industrial production process .
Chemical Reactions Analysis
Types of Reactions: Glycerol 1,2-dimethacrylate primarily undergoes polymerization reactions, particularly free radical polymerization. It can also participate in copolymerization with other monomers to form various polymeric materials .
Common Reagents and Conditions:
Polymerization: Initiators such as benzoyl peroxide or azobisisobutyronitrile (AIBN) are commonly used to initiate the free radical polymerization of this compound.
Copolymerization: this compound can be copolymerized with other monomers such as styrene, methyl methacrylate, or acrylates to form copolymers with tailored properties.
Major Products Formed: The primary products formed from the polymerization of this compound are cross-linked polymer networks. These networks exhibit enhanced mechanical strength, thermal stability, and chemical resistance, making them suitable for various applications .
Scientific Research Applications
Dental Materials
GDMA is primarily used as a cross-linking agent in dental resin systems. Its ability to form highly cross-linked polymer networks enhances the mechanical properties of dental composites, making them more durable and resistant to wear.
- Case Study : A study demonstrated that incorporating GDMA into dental resins significantly improved their stiffness and hardness compared to traditional materials .
Polymer Matrix Development
GDMA serves as a diluent in the formulation of polymer matrices alongside other methacrylate monomers such as diurethanedimethacrylate (DUDMA) and urethane dimethacrylate (UDMA). This combination is crucial for developing 3D-printable antimicrobial composite materials that can be utilized in various applications, including dentistry and tissue engineering.
- Research Insight : The synthesis of antimicrobial composites using GDMA has shown promising results in reducing bacterial colonization on dental surfaces .
Thermoresponsive Microbeads
The compound is also employed in synthesizing thermoresponsive microbeads with potential applications in drug delivery systems and tissue engineering. The cross-linking properties of GDMA contribute to the stability and responsiveness of these microbeads to temperature changes.
- Application Example : Research has indicated that microbeads created with GDMA can effectively encapsulate therapeutic agents, releasing them in a controlled manner when exposed to specific thermal conditions .
Comparative Data Table
Application Area | Description | Benefits |
---|---|---|
Dental Materials | Used as a cross-linking agent in resin systems | Enhanced durability and mechanical strength |
Polymer Matrices | Forms polymeric structures with other methacrylates | Development of antimicrobial composites |
Thermoresponsive Microbeads | Synthesis for drug delivery and tissue engineering | Controlled release of therapeutic agents |
Safety Considerations
Glycerol 1,2-dimethacrylate is classified with several hazard statements indicating skin irritation and serious eye irritation risks. Proper handling procedures must be followed to mitigate these risks:
- Hazard Statements :
- H315: Causes skin irritation.
- H319: Causes serious eye irritation.
Protective gear such as gloves and eye protection should be worn during handling .
Mechanism of Action
The mechanism of action of glycerol 1,2-dimethacrylate primarily involves its ability to undergo free radical polymerization. The methacrylate groups in the compound react with free radicals generated by initiators, leading to the formation of polymer chains. These chains further cross-link to form a three-dimensional network, imparting desirable properties to the resulting polymer .
Comparison with Similar Compounds
Key Properties:
- Molecular formula : C₁₀H₁₄O₅
- Functional groups : Two methacrylate groups (hydrophobic) and one hydroxyl group (hydrophilic) .
- Applications : Primarily used in dental adhesives (e.g., Adper Single Bond 2, containing 5–10 wt% GDMA) due to its ability to copolymerize with other methacrylates like BisGMA and HEMA .
- Safety : Classified as a skin and eye irritant (GHS Category 2/2A), requiring handling precautions .
Comparison with Similar Compounds
GDMA belongs to the family of dimethacrylate monomers, which are widely employed as crosslinkers in polymers. Below is a detailed comparison with structurally and functionally related compounds:
Structural and Functional Comparison
Notes:
- GDMA’s hydroxyl group enhances hydrophilicity, improving adhesion to moist substrates like dentin .
- Bis-GMA and GDMA are often combined in dental formulations to balance viscosity and reactivity .
Adhesive Strength:
- GDMA-containing adhesives (e.g., Adper Single Bond 2) exhibit dentin bond strengths comparable to commercial controls (~30–40 MPa) .
- Glycerol Phosphate Dimethacrylate (GPDM) , a phosphate-modified analog, shows superior dentin adhesion due to ionic interactions with calcium in dentin, but GDMA is more cost-effective .
Polymerization Efficiency:
- GDMA achieves >70% degree of conversion when photopolymerized with camphorquinone/amine systems, similar to EGDMA and TEGDMA .
- In Bis-GMA/glycerol systems, GDMA’s addition minimally affects thermal degradation profiles, maintaining stability up to 220°C .
Commercial Availability
Biological Activity
Glycerol 1,2-dimethacrylate (GDMA) is a hydrophilic dimethacrylate monomer that has garnered attention in various fields, particularly in dental materials and biomedical applications. This article explores GDMA's biological activity, its physicochemical properties, and its potential applications based on recent research findings.
Overview of this compound
GDMA is primarily used as a cross-linking agent in the formulation of dental adhesives and composite materials. Its structure allows for enhanced mechanical properties and improved polymerization compared to traditional monomers like hydroxyethyl methacrylate (HEMA). GDMA's dual methacrylate functionalities facilitate higher degrees of conversion during polymerization, which is crucial for the performance of dental materials.
The physicochemical properties of GDMA play a significant role in its biological activity. Key properties include:
- Molecular Weight : Approximately 200.24 g/mol.
- Density : 1.12 g/mL at 25 °C.
- Boiling Point : 120 °C at 1 mmHg.
- Refractive Index : .
These properties contribute to GDMA's effectiveness as a cross-linking agent in various applications.
Dental Applications
-
Adhesive Systems : GDMA has been studied as a replacement for HEMA in dental adhesives. Research indicates that GDMA enhances the mechanical properties of adhesives, leading to improved elastic modulus and bond strength. In a comparative study, GDMA-containing adhesives exhibited better water resistance and lower solubility than those containing HEMA, making them more suitable for clinical use .
Property HEMA Adhesive GDMA Adhesive Elastic Modulus (MPa) Lower Higher Water Sorption Higher Lower Solubility Higher Lower - Antimicrobial Properties : Recent studies have demonstrated the antimicrobial activity of GDMA-based polymers against common pathogens such as Escherichia coli and Staphylococcus aureus. This property is particularly valuable in dental applications where infection control is critical .
Biomedical Applications
GDMA's ability to form highly cross-linked networks makes it suitable for various biomedical applications, including:
- Drug Delivery Systems : The thermoresponsive properties of GDMA allow for the development of smart drug delivery systems that can release therapeutic agents in response to specific stimuli .
- Tissue Engineering : GDMA is utilized in creating scaffolds that mimic natural tissue structures, promoting cell attachment and growth.
Study on Adhesive Performance
A study conducted by Araújo-Neto et al. compared the bonding performance of simplified adhesives containing either HEMA or GDMA. The results showed that adhesives with GDMA not only achieved a higher degree of conversion but also demonstrated superior mechanical properties after aging under simulated pulpal pressure . The study utilized three-point bending tests and microtensile bond strength assessments to evaluate performance.
Antimicrobial Efficacy
In another study focusing on the antimicrobial properties of GDMA-based polymers, researchers synthesized photopolymers that exhibited significant antibacterial activity against E. coli and antifungal activity against Aspergillus niger. These findings suggest potential applications in developing antimicrobial coatings for medical devices .
Q & A
Basic Research Questions
Q. What are the established methods for synthesizing glycerol 1,2-dimethacrylate, and how do reaction conditions influence its purity and isomer distribution?
GDMA is typically synthesized via esterification of glycerol with methacrylic acid derivatives. Key factors include:
- Catalyst selection : Acid catalysts (e.g., sulfuric acid) or enzyme-mediated routes to control esterification specificity .
- Temperature and solvent : Reactions are often conducted at 60–80°C in aprotic solvents (e.g., dimethylformamide) to minimize side reactions .
- Isomer separation : GDMA exists as 1,2- and 1,3-isomers. Chromatographic techniques (HPLC or GC-MS) are required to resolve mixtures, as isomer ratios affect polymer properties .
Q. How is GDMA characterized structurally and functionally in polymer research?
- FTIR and NMR : Confirm methacrylate double bonds (C=C at 1635–1640 cm⁻¹ in FTIR) and ester linkages (δ 4.2–4.5 ppm in ¹H NMR) .
- Chromatography : HPLC or GPC evaluates monomer purity and residual inhibitors (e.g., MEHQ) .
- Thermal analysis : DSC identifies glass transition temperatures (Tg) and curing exotherms during photopolymerization .
Q. What role does GDMA play in dental resin formulations, and how does it enhance material performance?
GDMA acts as a hydrophilic crosslinker in dental adhesives and composites:
- Water diffusion : Enhances monomer penetration into moist dentin, improving bond strength .
- Mechanical properties : Increases crosslink density, improving microhardness (e.g., Knoop hardness ~40–50 MPa in polymerized resins) .
- Commercial formulations : Used at 5–10 wt% in adhesives like Adper Single Bond 2, alongside Bis-GMA and HEMA .
Advanced Research Questions
Q. How does glycerol content in GDMA-based polymers affect thermal stability and degradation kinetics?
Studies using TG-DTA and DTG show:
- Minimal thermal impact : Adding glycerol (≤20 wt%) to GDMA systems (e.g., UDMA/glycerol) does not significantly alter decomposition temperatures (~300–350°C) .
- Degradation mechanism : Two-stage mass loss: first from glycerol oxidation (200–250°C), then methacrylate backbone breakdown (>350°C) .
- Contradictions : Some studies report reduced char residue with glycerol, suggesting altered crosslinking efficiency .
Q. What experimental design challenges arise when optimizing GDMA for photopolymerization, and how can they be mitigated?
- Oxygen inhibition : Surface tackiness due to oxygen quenching radicals. Solutions include nitrogen purging or adding amine co-initiators (e.g., ethyl-p-dimethylaminobenzoate) .
- Curing depth : GDMA’s high viscosity limits light penetration. Diluents (e.g., TEGDMA) or ethanol (40 vol%) improve monomer mobility .
- Conversion efficiency : Use mid-IR spectroscopy to monitor methacrylate C=C conversion (~75–85% in optimized systems) .
Q. How do conflicting results on GDMA’s hydrophilicity versus durability in dental resins inform material design?
- Hydrophilicity benefit : GDMA improves wetting but increases water sorption, risking hydrolytic degradation .
- Balancing strategies :
- Nanofillers : Silica nanoparticles (10–20 wt%) reduce water uptake while maintaining bond strength .
- Hybrid monomers : Combining GDMA with hydrophobic UDMA improves aging resistance without compromising adhesion .
Q. What comparative advantages does GDMA offer over other dimethacrylates (e.g., TEGDMA, Bis-GMA) in polymer networks?
- Flexibility : GDMA’s glycerol backbone increases chain mobility vs. rigid Bis-GMA, reducing shrinkage stress .
- Biocompatibility : Lower cytotoxicity than HEMA-based systems due to reduced leachables .
- Limitations : Higher viscosity than TEGDMA requires solvent adjustments for clinical handling .
Q. Methodological Guidance
Q. How to analyze GDMA’s role in polymer morphology using SEM?
- Sample preparation : Cure GDMA resins in Teflon molds (2 mm thickness), polish, and sputter-coat with gold .
- Imaging : Use 5–10 kV accelerating voltage to resolve surface homogeneity. GDMA/glycerol systems show smoother morphologies vs. phase-separated Bis-GMA .
Q. What statistical approaches resolve contradictions in GDMA-based polymer performance data?
- Multivariate ANOVA : Compare mechanical properties (e.g., microtensile bond strength) across formulations with varying GDMA content .
- Accelerated aging tests : Soak samples in artificial saliva (37°C, 6 months) to correlate water sorption with long-term durability .
Properties
IUPAC Name |
[3-hydroxy-2-(2-methylprop-2-enoyloxy)propyl] 2-methylprop-2-enoate | |
---|---|---|
Source | PubChem | |
URL | https://pubchem.ncbi.nlm.nih.gov | |
Description | Data deposited in or computed by PubChem | |
InChI |
InChI=1S/C11H16O5/c1-7(2)10(13)15-6-9(5-12)16-11(14)8(3)4/h9,12H,1,3,5-6H2,2,4H3 | |
Source | PubChem | |
URL | https://pubchem.ncbi.nlm.nih.gov | |
Description | Data deposited in or computed by PubChem | |
InChI Key |
UKMBKKFLJMFCSA-UHFFFAOYSA-N | |
Source | PubChem | |
URL | https://pubchem.ncbi.nlm.nih.gov | |
Description | Data deposited in or computed by PubChem | |
Canonical SMILES |
CC(=C)C(=O)OCC(CO)OC(=O)C(=C)C | |
Source | PubChem | |
URL | https://pubchem.ncbi.nlm.nih.gov | |
Description | Data deposited in or computed by PubChem | |
Molecular Formula |
C11H16O5 | |
Source | PubChem | |
URL | https://pubchem.ncbi.nlm.nih.gov | |
Description | Data deposited in or computed by PubChem | |
Molecular Weight |
228.24 g/mol | |
Source | PubChem | |
URL | https://pubchem.ncbi.nlm.nih.gov | |
Description | Data deposited in or computed by PubChem | |
Synthesis routes and methods
Procedure details
Retrosynthesis Analysis
AI-Powered Synthesis Planning: Our tool employs the Template_relevance Pistachio, Template_relevance Bkms_metabolic, Template_relevance Pistachio_ringbreaker, Template_relevance Reaxys, Template_relevance Reaxys_biocatalysis model, leveraging a vast database of chemical reactions to predict feasible synthetic routes.
One-Step Synthesis Focus: Specifically designed for one-step synthesis, it provides concise and direct routes for your target compounds, streamlining the synthesis process.
Accurate Predictions: Utilizing the extensive PISTACHIO, BKMS_METABOLIC, PISTACHIO_RINGBREAKER, REAXYS, REAXYS_BIOCATALYSIS database, our tool offers high-accuracy predictions, reflecting the latest in chemical research and data.
Strategy Settings
Precursor scoring | Relevance Heuristic |
---|---|
Min. plausibility | 0.01 |
Model | Template_relevance |
Template Set | Pistachio/Bkms_metabolic/Pistachio_ringbreaker/Reaxys/Reaxys_biocatalysis |
Top-N result to add to graph | 6 |
Feasible Synthetic Routes
Disclaimer and Information on In-Vitro Research Products
Please be aware that all articles and product information presented on BenchChem are intended solely for informational purposes. The products available for purchase on BenchChem are specifically designed for in-vitro studies, which are conducted outside of living organisms. In-vitro studies, derived from the Latin term "in glass," involve experiments performed in controlled laboratory settings using cells or tissues. It is important to note that these products are not categorized as medicines or drugs, and they have not received approval from the FDA for the prevention, treatment, or cure of any medical condition, ailment, or disease. We must emphasize that any form of bodily introduction of these products into humans or animals is strictly prohibited by law. It is essential to adhere to these guidelines to ensure compliance with legal and ethical standards in research and experimentation.