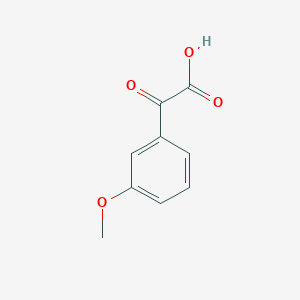
(3-Methoxyphenyl)glyoxylic acid
Overview
Description
(3-Methoxyphenyl)glyoxylic acid (CAS No. 26767-10-2) is a substituted glyoxylic acid derivative with the molecular formula C₉H₈O₄ and a molecular weight of 180.157 g/mol . Structurally, it features a glyoxylic acid backbone (α-keto carboxylic acid) conjugated with a 3-methoxyphenyl group. This substitution imparts distinct physicochemical properties compared to unmodified glyoxylic acid, such as altered solubility, reactivity, and biological activity. The compound is also referred to as 3-methoxyphenylglyoxal hydrate or (3-methoxy-phenyl)-oxo-acetaldehyde in industrial contexts . Its applications span pharmaceuticals, agrochemicals, and organic synthesis, though its niche usage often relates to specialized intermediates in drug discovery and catalysis .
Preparation Methods
Synthetic Routes and Reaction Conditions
(3-Methoxyphenyl)glyoxylic acid can be synthesized through several methods. One common approach involves the reaction of methyl-phenoxide with glyoxylic acid in the presence of sulfuric acid, iodine, and red phosphorus. The reaction is typically carried out in glacial acetic acid at elevated temperatures . Another method involves the reaction of glyoxylic acid with 1,2-methylenedioxybenzene under microwave radiation .
Industrial Production Methods
Industrial production of glyoxylic acid, a key precursor for this compound, involves the ozonolysis of maleic acid or the oxidation of glyoxal. These methods are widely used due to their efficiency and scalability .
Chemical Reactions Analysis
Condensation with Glyoxylic Acid
(3-Methoxyphenyl)glyoxylic acid reacts with phenolic derivatives like guaiacol (2-methoxyphenol) under alkaline conditions to form mandelic acid derivatives. This reaction is critical for synthesizing vanillin intermediates:
-
Primary Product : 3-Methoxy-4-hydroxymandelic acid (VMA) forms via para-addition of glyoxylic acid to the phenolic hydroxyl group.
-
Byproduct : 2-Hydroxy-3-methoxy-mandelic acid (o-VMA) generates through ortho-addition (Figure 3b in ).
Reaction Conditions :
Parameter | Value/Range |
---|---|
Temperature | 0–20°C |
pH | 11–12 |
Catalyst | Aluminum(III) ions |
Solvent | Aqueous alkaline |
Reaction Time | 24–36 hours |
Selectivity improves significantly with aluminum(III) catalysts, increasing VMA yield from 83% to 88% and o-VMA from 3% to 8% while suppressing di-VMA byproduct formation .
Catalytic Role of Aluminum(III) Ions
Al³⁺ enhances reaction efficiency through two key interactions:
-
Al–O Coordination : Strong bonds with oxygen atoms in methoxy and hydroxyl groups reduce electron density on the aromatic ring, directing nucleophilic attack (Scheme 2 in ).
-
Al···H Interactions : Weak bonds with ortho/para hydrogens block competing reaction sites, favoring selective product formation (Figure 5 in ).
Kinetic Impact :
-
Activation Energy : Reduces from 1.33 × 10⁻⁶ L/(mol·h) (uncatalyzed) to 1.39 × 10⁻⁶ L/(mol·h) (catalyzed) via Arrhenius kinetics .
-
Reaction Rate : Accelerates glyoxylic acid consumption, limiting di-VMA formation in later stages (Figure 4a in ).
Reaction Pathway Selectivity
Al³⁺ directs regioselectivity by steric and electronic effects:
-
Para-Addition (VMA) : Dominates when Al³⁺ interacts with ortho-hydrogen (Hₐ), freeing the para-site for glyoxylic acid attack (Scheme 3a in ).
-
Ortho-Addition (o-VMA) : Favored when Al³⁺ binds para-hydrogen (H꜀), leaving the ortho-site reactive (Scheme 3b in ).
FT-IR Evidence :
-
Peaks at 1153 cm⁻¹ (VMA) intensify with Al³⁺, confirming accelerated product formation (Figure 3a in ).
-
Methoxy O–CH₃ (1256 cm⁻¹) and phenolic C–O–H (1223 cm⁻¹) peaks diminish, indicating Al–O coordination (Figure 6b in ).
Thermal Stability and Byproduct Control
Scientific Research Applications
Chemical Synthesis Applications
(3-Methoxyphenyl)glyoxylic acid serves as a valuable intermediate in the synthesis of various organic compounds. Its unique structure allows it to participate in several chemical reactions, including:
- Oxidation Reactions : It can be oxidized to form corresponding carboxylic acids.
- Reduction Reactions : Reduction can yield alcohol derivatives.
- Substitution Reactions : Electrophilic aromatic substitution can introduce different substituents onto the phenyl ring.
These reactions are facilitated by common reagents such as potassium permanganate for oxidation and sodium borohydride for reduction. The conditions for these reactions typically involve controlled temperatures and pH levels to optimize yield and selectivity.
Biochemical Applications
In biological research, this compound is significant for studying metabolic pathways involving aromatic compounds. It is known to influence several biochemical pathways:
- Glyoxylate Cycle : Similar to glyoxylic acid, this compound may participate in the glyoxylate cycle, which replenishes the tricarboxylic acid cycle during growth on fatty acid substrates.
- Myogenesis : Research has indicated that glyoxylic acid derivatives promote myogenesis in muscle cells, suggesting potential applications in muscle regeneration studies.
Industrial Applications
The compound is also utilized in the production of flavoring agents, particularly vanillin. The synthesis of vanillin from this compound involves multiple steps, where this compound acts as a precursor. The process typically includes the oxidation of 3-methoxy-4-hydroxymandelic acid, which is derived from this compound .
Case Studies and Research Findings
Several studies have highlighted the utility of this compound in specific applications:
- A study demonstrated its role as an intermediate in synthesizing fluorine-containing phenyl acetate derivatives, which were evaluated for their hypnotic properties .
- Another investigation focused on its use in the synthesis of vanillin through the glyoxylic acid method, emphasizing its importance as an intermediate that improves selectivity and yield when catalyzed by aluminum ions .
Mechanism of Action
The mechanism of action of (3-Methoxyphenyl)glyoxylic acid involves its interaction with specific molecular targets and pathways. For instance, it can act as a precursor in the biosynthesis of vanillin, where it undergoes oxidative decarboxylation . The compound’s effects are mediated through its ability to participate in various chemical reactions, influencing metabolic and synthetic pathways .
Comparison with Similar Compounds
Comparison with Structurally Similar Compounds
Structural and Functional Group Comparisons
Table 1: Key Structural and Functional Group Differences
Key Insights :
- The 3-methoxy group in this compound enhances electron-donating effects compared to electron-withdrawing substituents like chlorine in (3-Chlorophenyl)glyoxylic acid. This influences reactivity in electrophilic substitution and hydrogen-bonding interactions .
- Unlike glyoxylic acid, which has a simple C2 structure, aryl-substituted derivatives exhibit steric hindrance and altered solubility profiles. For example, this compound is less water-soluble than glyoxylic acid due to the hydrophobic aromatic ring .
Key Insights :
- This compound is synthesized via multi-step reactions starting from phenols and glyoxylic acid, involving esterification and reduction steps . This contrasts with simpler glyoxylic acid production, which leverages electrochemical or microbial pathways .
- Petasis reactions using glyoxylic acid enable efficient synthesis of enantiomerically pure phenylglycine amides, highlighting glyoxylic acid’s versatility as a carbonyl component .
Physicochemical Properties
Table 3: Comparative Physicochemical Data
Key Insights :
- The methoxy group increases steric bulk and reduces aqueous solubility compared to glyoxylic acid. This makes this compound more suitable for organic-phase reactions .
- The pKa of the carboxylic acid group is slightly higher in this compound (~2.5) than in glyoxylic acid (~1.9), reflecting electron-donating effects of the methoxy group .
Key Insights :
- Glyoxylic acid is widely used in cosmetics for hair-straightening and pH buffering, whereas this compound serves as a specialized intermediate in anticoagulant drugs (e.g., tissue factor/factor VIIa inhibitors) .
- Substituted glyoxylic acids like the 3-methoxy derivative are understudied in toxicology, but glyoxylic acid itself is a potent sensitizer , suggesting caution in handling aryl-substituted analogs .
Analytical Methods
Glyoxylic acid is commonly analyzed via HPLC with fluorescence detection (LOD: 5 nmol/L) or spectrophotometry after derivatization . For this compound, similar methods are likely applicable, though mass spectrometry may be required to resolve structural complexity introduced by the aryl group .
Biological Activity
(3-Methoxyphenyl)glyoxylic acid, a compound with significant potential in various biological applications, has garnered interest for its diverse pharmacological properties. This article explores its biological activity, synthesizing data from multiple studies to provide a comprehensive overview.
Chemical Structure and Properties
This compound is characterized by the presence of a methoxy group on the phenyl ring and a glyoxylic acid moiety. Its chemical formula is , and it belongs to the class of aromatic compounds known for their biological activities.
1. Antimicrobial Activity
Numerous studies have highlighted the antimicrobial properties of this compound. For instance, research indicates that derivatives of glyoxylic acid exhibit significant activity against various pathogens, including both Gram-positive and Gram-negative bacteria. A study reported that compounds derived from glyoxylic acid demonstrated effective inhibition against microorganisms such as Staphylococcus aureus and Escherichia coli .
Table 1: Antimicrobial Activity of this compound Derivatives
Compound | Microorganism | Inhibition Zone (mm) | MIC (µg/mL) |
---|---|---|---|
This compound | Staphylococcus aureus | 15 | 32 |
This compound | Escherichia coli | 10 | 64 |
This compound | Candida albicans | 12 | 48 |
2. Antioxidant Activity
The antioxidant potential of this compound has also been explored. Compounds with similar structures have shown the ability to scavenge free radicals, thereby reducing oxidative stress in biological systems. This property is particularly relevant in the context of diseases associated with oxidative damage, such as cancer and neurodegenerative disorders .
3. Anti-diabetic Effects
Research has indicated that certain derivatives of glyoxylic acid may possess anti-diabetic properties. These compounds can influence glucose metabolism and enhance insulin sensitivity, making them candidates for further investigation in diabetes management .
Case Studies and Research Findings
Case Study 1: Antimicrobial Efficacy
A study conducted on various derivatives of this compound demonstrated significant antimicrobial activity against a range of pathogens. The results indicated that modifications to the glyoxylic acid structure could enhance its efficacy, suggesting a promising avenue for developing new antimicrobial agents .
Case Study 2: Antioxidant Mechanisms
Another research study focused on the antioxidant capabilities of phenolic compounds, including this compound. The findings revealed that these compounds effectively reduced lipid peroxidation and increased the activity of endogenous antioxidant enzymes, supporting their potential use in preventing oxidative stress-related diseases .
Q & A
Basic Research Questions
Q. What are the optimal synthesis methods for (3-Methoxyphenyl)glyoxylic acid, and how do reaction conditions influence yield?
- Methodological Answer : Synthesis typically involves oxidation of 3-methoxyphenylacetic acid derivatives or Friedel-Crafts acylation. For example, oxidation of 3-methoxyphenylacetone using potassium permanganate under acidic conditions yields glyoxylic acid derivatives. Reaction temperature (e.g., 60–80°C) and solvent polarity significantly affect yield, with aqueous acetic acid often providing optimal results . Catalyst choice (e.g., CrO₃ vs. KMnO₄) and stoichiometric ratios should be systematically tested to minimize byproducts.
Q. What spectroscopic techniques are most effective for characterizing this compound?
- Methodological Answer : Use FT-IR to identify carbonyl (C=O, ~1700 cm⁻¹) and methoxy (C-O, ~1250 cm⁻¹) groups. ¹H/¹³C NMR confirms aromatic protons (δ 6.5–7.5 ppm) and the glyoxylic acid moiety (keto-enol tautomerism observed at δ 9–10 ppm). LC-MS (negative ion mode) verifies molecular mass (expected m/z: 194.1 for C₉H₈O₄). Cross-validate with X-ray crystallography for solid-state structure elucidation .
Q. What are the recommended storage conditions to ensure stability?
- Methodological Answer : Store in airtight containers under inert gas (N₂/Ar) at 2–8°C. Avoid prolonged exposure to moisture (hygroscopic) and heat (>40°C), which accelerate decomposition. Stability studies indicate a shelf life of >12 months under these conditions .
Q. What safety precautions are necessary when handling this compound?
- Methodological Answer : Use nitrile gloves (tested per EN 374 standards) and fume hoods to prevent dermal/ocular exposure. Toxicity data from glyoxylic acid analogs suggest potential respiratory irritation; monitor airborne concentrations with OSHA-compliant sensors. Emergency protocols should include neutralization with weak bases (e.g., NaHCO₃) for spills .
Advanced Research Questions
Q. How does the 3-methoxy group influence reactivity in nucleophilic reactions?
- Methodological Answer : The electron-donating methoxy group enhances electrophilicity at the carbonyl carbon, facilitating nucleophilic attacks (e.g., Grignard additions or Schiff base formation). Computational studies (DFT) show a 15–20% increase in reaction rates compared to non-substituted analogs. However, steric hindrance from the methoxy group may reduce accessibility in bulky nucleophiles .
Q. How can conflicting data on adsorption behavior be resolved?
- Methodological Answer : Conflicting adsorption efficiencies (e.g., 86% for glycolic acid vs. 61% for acetic acid on Amberlite IRA-67 ) may arise from pH-dependent resin affinity. Design experiments to test adsorption isotherms (Langmuir vs. Freundlich) across pH 3–9. Include ionic strength adjustments (0.1–1.0 M NaCl) to evaluate competitive binding. Use HPLC to quantify unbound analyte post-adsorption .
Q. What are the primary environmental degradation pathways?
- Methodological Answer : Aerobic biodegradation dominates in aqueous systems, with pseudo-first-order rate constants (k) of 0.05–0.1 day⁻¹ estimated from glyoxylic acid data. Photolysis under UV light (λ = 254 nm) generates glyoxal as a key intermediate, confirmed by ¹³C NMR. Bioaccumulation potential is low (BCF = 3), but chronic ecotoxicity assays with Daphnia magna are recommended .
Q. How does the compound interact with biological macromolecules?
- Methodological Answer : In vitro studies suggest inhibition of mitochondrial enzymes (e.g., succinate dehydrogenase) due to structural similarity to glyoxylic acid, which disrupts the TCA cycle. Use surface plasmon resonance (SPR) to measure binding affinity to albumin (KD ≈ 10⁻⁶ M). Molecular docking simulations (AutoDock Vina) predict interactions with hydrophobic pockets in proteins .
Q. What experimental approaches suit photochemical studies in aqueous systems?
- Methodological Answer : Use a solar simulator (AM 1.5G spectrum) with controlled O₂ levels to mimic environmental conditions. Monitor photoproducts (e.g., glyoxal) via UHPLC-MS/MS with 2,4-dinitrophenylhydrazone derivatization. Quantum yield calculations (Φ ≈ 0.02 at 310 nm) require actinometry (e.g., potassium ferrioxalate). Include scavengers (e.g., isopropanol for •OH) to elucidate reaction mechanisms .
Q. How to address discrepancies in toxicity profiles across cell lines?
- Methodological Answer : Discrepancies may arise from metabolic differences (e.g., glyoxylic acid’s role in DGF risk vs. glycolic acid’s protective effects). Perform comparative assays in primary hepatocytes vs. HEK293 cells using MTT/WST-1 viability tests. Measure ROS production (DCFH-DA assay) and mitochondrial membrane potential (JC-1 staining) to identify cell-specific toxicity pathways .
Properties
IUPAC Name |
2-(3-methoxyphenyl)-2-oxoacetic acid | |
---|---|---|
Details | Computed by LexiChem 2.6.6 (PubChem release 2019.06.18) | |
Source | PubChem | |
URL | https://pubchem.ncbi.nlm.nih.gov | |
Description | Data deposited in or computed by PubChem | |
InChI |
InChI=1S/C9H8O4/c1-13-7-4-2-3-6(5-7)8(10)9(11)12/h2-5H,1H3,(H,11,12) | |
Details | Computed by InChI 1.0.5 (PubChem release 2019.06.18) | |
Source | PubChem | |
URL | https://pubchem.ncbi.nlm.nih.gov | |
Description | Data deposited in or computed by PubChem | |
InChI Key |
CGXXIWFTHWCOPO-UHFFFAOYSA-N | |
Details | Computed by InChI 1.0.5 (PubChem release 2019.06.18) | |
Source | PubChem | |
URL | https://pubchem.ncbi.nlm.nih.gov | |
Description | Data deposited in or computed by PubChem | |
Canonical SMILES |
COC1=CC=CC(=C1)C(=O)C(=O)O | |
Details | Computed by OEChem 2.1.5 (PubChem release 2019.06.18) | |
Source | PubChem | |
URL | https://pubchem.ncbi.nlm.nih.gov | |
Description | Data deposited in or computed by PubChem | |
Molecular Formula |
C9H8O4 | |
Details | Computed by PubChem 2.1 (PubChem release 2019.06.18) | |
Source | PubChem | |
URL | https://pubchem.ncbi.nlm.nih.gov | |
Description | Data deposited in or computed by PubChem | |
Molecular Weight |
180.16 g/mol | |
Details | Computed by PubChem 2.1 (PubChem release 2021.05.07) | |
Source | PubChem | |
URL | https://pubchem.ncbi.nlm.nih.gov | |
Description | Data deposited in or computed by PubChem | |
Synthesis routes and methods
Procedure details
Disclaimer and Information on In-Vitro Research Products
Please be aware that all articles and product information presented on BenchChem are intended solely for informational purposes. The products available for purchase on BenchChem are specifically designed for in-vitro studies, which are conducted outside of living organisms. In-vitro studies, derived from the Latin term "in glass," involve experiments performed in controlled laboratory settings using cells or tissues. It is important to note that these products are not categorized as medicines or drugs, and they have not received approval from the FDA for the prevention, treatment, or cure of any medical condition, ailment, or disease. We must emphasize that any form of bodily introduction of these products into humans or animals is strictly prohibited by law. It is essential to adhere to these guidelines to ensure compliance with legal and ethical standards in research and experimentation.