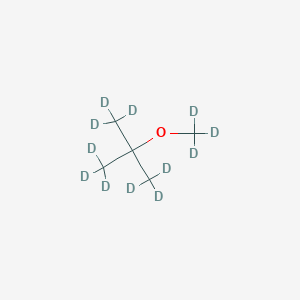
tert-Butyl methyl ether-d12
Overview
Description
tert-Butyl methyl ether-d12 ((CD₃)₃COCD₃) is a fully deuterated isotopologue of methyl tert-butyl ether (MTBE), where all hydrogen atoms are replaced with deuterium (²H or D). This compound is primarily utilized as a stable isotopic tracer in nuclear magnetic resonance (NMR) spectroscopy, reaction mechanism studies, and pharmacokinetic research due to its negligible proton interference and distinct spectral properties . Key characteristics include:
Preparation Methods
Synthetic Routes and Reaction Conditions
tert-Butyl methyl ether-d12 can be synthesized through the Williamson ether synthesis. This method involves the reaction of tert-butoxide ion with iodomethane-d3 under anhydrous conditions . The reaction proceeds as follows:
(CD3)3CO−+CD3I→(CD3)3COCD3+I−
The reaction is typically carried out in an inert atmosphere to prevent moisture from interfering with the reaction.
Industrial Production Methods
Industrial production of this compound involves the same principles as the laboratory synthesis but on a larger scale. The process requires high-purity deuterated reagents and stringent control of reaction conditions to ensure the isotopic purity of the final product.
Chemical Reactions Analysis
Acid-Catalyzed Hydrolysis
MTBE-d12 undergoes hydrolysis in acidic conditions to form deuterated tert-butyl alcohol (TBA-d9) and methanol-d3. This reaction follows second-order kinetics, with a rate constant () of approximately at 26°C . The mechanism involves protonation of the ether oxygen, followed by nucleophilic attack by water.
Hydrolysis Products Comparison:
Compound | Products | Rate Constant (26°C) |
---|---|---|
MTBE | TBA + Methanol | |
MTBE-d12 | TBA-d9 + Methanol-d3 | Slightly reduced |
Kinetic isotope effects (KIEs) lower the reaction rate due to stronger C-D bonds compared to C-H .
Thermal Decomposition
At elevated temperatures (>100°C), MTBE-d12 decomposes to isobutene-d6 and methanol-d3 via acid-catalyzed cracking. This reaction is exploited industrially for isobutene production :
Deuterium labeling confirms that the methyl group adjacent to the ether oxygen remains intact during cleavage, consistent with carbocation intermediacy .
Catalytic Methoxycarbonylation
MTBE-d12 participates in palladium-catalyzed methoxycarbonylation reactions to form deuterated methyl propanoate (D-methyl propanoate). Studies using Pd/1,2-DTBPMB catalysts reveal a hydride mechanism involving:
-
Deuterium transfer to palladium.
-
Reversible ethene coordination and migration.
Isotopomer Distribution in Methyl Propanoate:
Reaction Conditions | Dominant Isotopomer | Mechanism Evidence |
---|---|---|
CO-limited | D-methyl | Hydride pathway |
Non-CO-limited | D-methyl | Carbomethoxy pathway |
Oxidative Degradation
MTBE-d12 is resistant to ambient oxidation but degrades under advanced oxidation processes (AOPs). Fenton’s reagent (Fe/HO) achieves 90% degradation at pH 4, producing deuterated intermediates like tert-butyl formate-d9 and acetone-d6 .
Degradation Byproducts:
Process | Major Byproducts | Efficiency (%) |
---|---|---|
Acid Hydrolysis | TBA-d9, Methanol-d3 | ~90 |
Fenton’s Reaction | Acetone-d6, Formate-d9 | 90 |
Key Research Findings:
Scientific Research Applications
tert-Butyl methyl ether-d12 is widely used in scientific research due to its isotopic labeling. Some of its applications include:
Nuclear Magnetic Resonance (NMR) Spectroscopy: Used as a solvent or internal standard in NMR spectroscopy to study deuterium-labeled compounds.
Mass Spectrometry: Employed in mass spectrometry for the analysis of deuterium-labeled compounds.
Chemical Kinetics: Used in studies of reaction mechanisms and kinetics involving deuterium-labeled compounds.
Environmental Science: Applied in the study of environmental pollutants and their degradation pathways.
Mechanism of Action
The mechanism of action of tert-Butyl methyl ether-d12 is primarily related to its role as a solvent or reagent in various chemical reactions. Its deuterium atoms replace hydrogen atoms, which can significantly alter the reaction kinetics and mechanisms due to the isotope effect. This makes it a valuable tool in studying reaction pathways and mechanisms.
Comparison with Similar Compounds
Comparison with Similar Compounds
Methyl tert-Butyl Ether (MTBE)
Molecular Formula : C₅H₁₂O .
Key Properties :
Property | MTBE | MTBE-d12 |
---|---|---|
Molecular Weight | 88.15 g/mol | 100.22 g/mol |
Boiling Point | 55.2°C | Slightly higher* |
Density (20°C) | 0.740 g/cm³ | ~0.78 g/cm³† |
Flash Point | -28°C | Similar flammability |
Primary Use | Solvent, fuel additive | Isotopic tracer |
Functional Differences :
- Isotopic Effects : MTBE-d12 exhibits reduced proton NMR signal interference, making it ideal for mechanistic studies .
- Reactivity : Deuterium substitution may slow reaction kinetics (kinetic isotope effect) in processes involving C-H bond cleavage .
- Cost: MTBE-d12 is significantly more expensive (e.g., 500 mg costs ~JPY 83,600) compared to non-deuterated MTBE .
tert-Butyl Ethyl Ether
Molecular Formula : C₆H₁₄O .
Key Properties :
Property | tert-Butyl Ethyl Ether | MTBE-d12 |
---|---|---|
Molecular Weight | 102.18 g/mol | 100.22 g/mol |
Boiling Point | 72–74°C | ~55°C (est.) |
Structure | (CH₃)₃COCH₂CH₃ | (CD₃)₃COCD₃ |
Primary Use | Solvent, organic synthesis | Isotopic studies |
Functional Differences :
- Branching vs. Chain Length : The ethyl group in tert-butyl ethyl ether increases boiling point and lipophilicity compared to MTBE-d12 .
- Deuterated Analog: No deuterated form of tert-butyl ethyl ether is mentioned in the evidence, limiting direct isotopic comparisons.
tert-Butyl Acetate-d12
Molecular Formula : C₆D₁₂O₂ .
Key Properties :
Property | tert-Butyl Acetate-d12 | MTBE-d12 |
---|---|---|
Molecular Weight | 128.23 g/mol | 100.22 g/mol |
Functional Group | Ester (acetate) | Ether |
Primary Use | Isotopic labeling | NMR studies |
Functional Differences :
- Reactivity : The ester group in tert-butyl acetate-d12 is prone to hydrolysis, unlike the ether linkage in MTBE-d12 .
- Applications : tert-Butyl acetate-d12 is used in environmental tracer studies, while MTBE-d12 is favored in synthetic chemistry .
NMR Spectroscopy
- MTBE-d12: Reduces proton background noise in ¹H NMR, enabling precise analysis of dynamic molecular processes (e.g., tert-butyl group rotation in 2,4- and 2,5-ditert-butyl hydroxybenzenes) .
- Comparison with Non-Deuterated Ethers: MTBE-d12 provides clearer spectral data compared to tert-butyl ethyl ether, which requires deuterated solvents for high-resolution NMR .
Precautions :
Biological Activity
Introduction
tert-Butyl methyl ether-d12 (TBME-d12) is a deuterated form of tert-butyl methyl ether, a compound primarily used as a solvent and in organic synthesis. Understanding its biological activity is crucial due to its potential exposure risks and implications for human health. This article explores the pharmacokinetics, toxicological effects, and metabolic pathways associated with TBME-d12, highlighting key research findings and case studies.
Absorption and Elimination
Research indicates that TBME-d12 can be absorbed through various routes, including dermal, oral, and inhalation exposure. A study involving controlled exposures revealed that blood concentrations of TBME peaked shortly after exposure, with elimination kinetics described by a three-compartment model. The mean residence times for uptake and decay phases were significantly longer than those observed for other compounds like chloroform, suggesting a unique absorption profile for TBME-d12 .
Case Study: Dermal Exposure
In a controlled study, volunteers were exposed to TBME-d12 in bath water at a concentration of 150 µg/L. Breath analysis showed a gradual increase in breath concentrations, peaking at levels significantly higher than pre-exposure values. The study estimated that the mean amount of TBME-d12 exhaled was approximately 3.0 ± 1.1 µg after exposure . This suggests that while dermal absorption occurs, it is relatively lower compared to other routes.
Exposure Route | Peak Blood Concentration | Elimination Half-life | Mean Exhaled Amount |
---|---|---|---|
Dermal | Immediate | 41.5 ± 26.3 min | 3.0 ± 1.1 µg |
Oral | 15-30 min | Varies | Not specified |
Inhalation | End of exposure | Varies | Not specified |
Cellular Impact
TBME has been shown to exert toxic effects on various cell lines. A comparative study on rat fibroblast cells indicated that both TBME and its metabolite, tertiary butyl alcohol (TBA), inhibited cell growth but through different mechanisms. TBME caused an accumulation of cells in the S-phase of the cell cycle, while TBA led to G0/G1-phase accumulation, indicating distinct pathways of cellular toxicity .
Endocrine Disruption Potential
TBME is classified as an endocrine disruptor based on available in vitro evidence. It has demonstrated biological activity related to endocrine disruption in laboratory settings, raising concerns about its long-term effects on human health and wildlife .
Endpoint | TBME Effect | TBA Effect |
---|---|---|
Cell Cycle Progression | Accumulation in S-phase | Accumulation in G0/G1-phase |
DNA Damage | Induces oxidative damage | Induces oxidative damage |
Endocrine Disruption Evidence | Yes | Not specified |
Metabolism
The metabolism of TBME-d12 involves conversion to TBA, which has been identified as a significant metabolite following exposure. Studies suggest that oral exposure leads to greater metabolism into TBA compared to dermal or inhalation routes due to first-pass metabolism effects . The presence of TBA in blood samples can complicate the assessment of TBME exposure due to its common occurrence in consumer products.
Q & A
Basic Research Questions
Q. How is tert-butyl methyl ether-d12 synthesized in the laboratory?
Methodological Answer: The synthesis typically involves deuteration of the methyl and tert-butyl groups via catalytic exchange reactions or selective deuteration using deuterated precursors. For example, deuterated methanol (CD3OH) and tert-butanol-d12 can be reacted under acidic conditions to yield the fully deuterated ether. Purity is ensured through distillation and verified via mass spectrometry (MS) or nuclear magnetic resonance (NMR) to confirm ≥99 atom% deuterium incorporation .
Q. What safety protocols are essential when handling this compound in laboratory settings?
Methodological Answer: Due to its volatility and flammability (flash point: -28°C), use explosion-proof equipment and ensure proper ventilation. Avoid skin contact by wearing nitrile gloves and lab coats. In case of spills, employ inert adsorbents (e.g., vermiculite) and avoid ignition sources. Emergency showers and eye-wash stations must be accessible. Contaminated clothing should be laundered separately to prevent cross-exposure .
Q. What analytical techniques are recommended for quantifying this compound in reaction mixtures?
Methodological Answer: Gas chromatography (GC) with flame ionization detection (FID) or mass spectrometry (MS) is preferred for quantification. Deuterated compounds exhibit distinct mass-to-charge (m/z) ratios in MS (e.g., molecular ion at m/z 100 for C5D12O). For isotopic purity assessment, high-resolution NMR (e.g., <sup>2</sup>H NMR) can confirm deuterium distribution .
Advanced Research Questions
Q. How does isotopic labeling with deuterium in this compound influence its reaction kinetics compared to the non-deuterated analog?
Methodological Answer: Deuterium introduces kinetic isotope effects (KIEs), slowing reaction rates in bond-breaking steps (e.g., SN2 reactions). For example, cleavage of the C-O bond in acidic hydrolysis may show a KIE of 2–3 due to reduced zero-point energy in C-D vs. C-H bonds. Isotopic tracing via GC-MS can monitor deuterium retention in products to elucidate mechanisms .
Q. What are the challenges in tracking environmental degradation pathways of deuterated ethers like this compound using advanced oxidation processes (AOPs)?
Methodological Answer: AOPs (e.g., UV/H2O2/Fe(II)) generate hydroxyl radicals that degrade ethers. Deuterated analogs may exhibit altered reactivity due to KIEs, requiring adjusted rate constants. LC-MS/MS with isotopic resolution is critical to distinguish degradation intermediates (e.g., deuterated formaldehyde or acetone) from background signals in environmental matrices .
Q. How can NMR spectroscopy be optimized to distinguish this compound from its protonated counterpart in complex mixtures?
Methodological Answer: <sup>13</sup>C NMR detects isotopic shifts in carbon environments (e.g., CD3 vs. CH3 groups). For <sup>1</sup>H NMR, suppression of residual proton signals is achieved using deuterium decoupling. 2D heteronuclear single-quantum coherence (HSQC) can map deuterium-carbon correlations, enabling precise identification in multicomponent systems .
Properties
IUPAC Name |
1,1,1,3,3,3-hexadeuterio-2-(trideuteriomethoxy)-2-(trideuteriomethyl)propane | |
---|---|---|
Details | Computed by LexiChem 2.6.6 (PubChem release 2019.06.18) | |
Source | PubChem | |
URL | https://pubchem.ncbi.nlm.nih.gov | |
Description | Data deposited in or computed by PubChem | |
InChI |
InChI=1S/C5H12O/c1-5(2,3)6-4/h1-4H3/i1D3,2D3,3D3,4D3 | |
Details | Computed by InChI 1.0.5 (PubChem release 2019.06.18) | |
Source | PubChem | |
URL | https://pubchem.ncbi.nlm.nih.gov | |
Description | Data deposited in or computed by PubChem | |
InChI Key |
BZLVMXJERCGZMT-MGKWXGLJSA-N | |
Details | Computed by InChI 1.0.5 (PubChem release 2019.06.18) | |
Source | PubChem | |
URL | https://pubchem.ncbi.nlm.nih.gov | |
Description | Data deposited in or computed by PubChem | |
Canonical SMILES |
CC(C)(C)OC | |
Details | Computed by OEChem 2.1.5 (PubChem release 2019.06.18) | |
Source | PubChem | |
URL | https://pubchem.ncbi.nlm.nih.gov | |
Description | Data deposited in or computed by PubChem | |
Isomeric SMILES |
[2H]C([2H])([2H])C(C([2H])([2H])[2H])(C([2H])([2H])[2H])OC([2H])([2H])[2H] | |
Details | Computed by OEChem 2.1.5 (PubChem release 2019.06.18) | |
Source | PubChem | |
URL | https://pubchem.ncbi.nlm.nih.gov | |
Description | Data deposited in or computed by PubChem | |
Molecular Formula |
C5H12O | |
Details | Computed by PubChem 2.1 (PubChem release 2019.06.18) | |
Source | PubChem | |
URL | https://pubchem.ncbi.nlm.nih.gov | |
Description | Data deposited in or computed by PubChem | |
Molecular Weight |
100.22 g/mol | |
Details | Computed by PubChem 2.1 (PubChem release 2021.05.07) | |
Source | PubChem | |
URL | https://pubchem.ncbi.nlm.nih.gov | |
Description | Data deposited in or computed by PubChem | |
Disclaimer and Information on In-Vitro Research Products
Please be aware that all articles and product information presented on BenchChem are intended solely for informational purposes. The products available for purchase on BenchChem are specifically designed for in-vitro studies, which are conducted outside of living organisms. In-vitro studies, derived from the Latin term "in glass," involve experiments performed in controlled laboratory settings using cells or tissues. It is important to note that these products are not categorized as medicines or drugs, and they have not received approval from the FDA for the prevention, treatment, or cure of any medical condition, ailment, or disease. We must emphasize that any form of bodily introduction of these products into humans or animals is strictly prohibited by law. It is essential to adhere to these guidelines to ensure compliance with legal and ethical standards in research and experimentation.