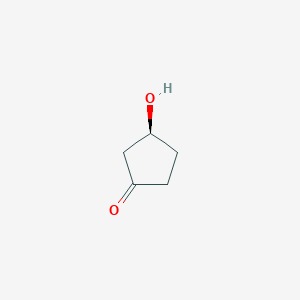
3-Hydroxycyclopentanone
- Click on QUICK INQUIRY to receive a quote from our team of experts.
- With the quality product at a COMPETITIVE price, you can focus more on your research.
Overview
Description
3-Hydroxycyclopentanone is a chemical compound with the molecular formula C5H8O2 . It is a key intermediate in various chemical reactions and has potential applications in different fields .
Synthesis Analysis
The synthesis of 3-Hydroxycyclopentanone involves complex chemical reactions. For instance, one study describes the preparation of enantiomerically enriched ®-3-hydroxycyclopentanone . Another research paper discusses the semirational engineering of a thermostable carbonyl reductase for the precision synthesis of (2R,3R)-2-Methyl-2-benzyl-3-hydroxycyclopentanone and its analogues .Molecular Structure Analysis
The molecular structure of 3-Hydroxycyclopentanone is characterized by a five-membered ring with a ketone and a hydroxyl group. The molecular weight is 100.116 Da and the monoisotopic mass is 100.052429 Da .Physical And Chemical Properties Analysis
3-Hydroxycyclopentanone has a density of 1.2±0.1 g/cm3, a boiling point of 210.0±33.0 °C at 760 mmHg, and a vapor pressure of 0.0±0.9 mmHg at 25°C . It has 2 hydrogen bond acceptors, 1 hydrogen bond donor, and no freely rotating bonds .Scientific Research Applications
Enantioselective Preparation
- Enantioselective Synthesis : A method for preparing enantiomerically enriched (R) and (S)-3-hydroxycyclopentanone was developed using kinetic resolution. This process involved the use of Pseudomonas cepacia lipase, indicating its potential in producing chiral intermediates for pharmaceutical and other industries (Chen & Hanefeld, 2013).
Biomass Conversion
- Biomass-derived Intermediates : The conversion of biomass-based 5-hydroxymethyl furfural (5-HMF) into 3-hydroxymethylcyclopentanone (HCPN), a derivative of 3-hydroxycyclopentanone, was achieved using a Ni-Fe/Al2O3 catalyst. This highlights its role in synthesizing polymers, pesticides, and fragrances from renewable sources (Li et al., 2021).
Chemical Synthesis
- Intramolecular Pauson–Khand Reaction : A novel substrate-specific formation of 3-hydroxycyclopentanone was discovered during the annulation of a 3-methylcyclopentenone moiety onto a cyclopentenol double bond. This finding suggests its utility in complex chemical syntheses (Muto & Ogasawara, 2001).
Pharmaceutical Research
- Alzheimer's Disease Research : Studies on cyclopentanone derivatives, including compounds related to 3-hydroxycyclopentanone, showed potential therapeutic effects for Alzheimer's disease. This includes reducing oxidative stress and neuroinflammation in animal models (Ullah et al., 2020).
Organic Synthesis
- Natural Product Isolation : The isolation of compounds such as 2-hydroxymethyl-3-methylcyclopentanone from endophytic fungi indicates the potential of 3-hydroxycyclopentanone derivatives in the field of organic synthesis and natural product research (Chomcheon et al., 2006).
Mechanism of Action
Target of Action
It’s known that cyclic β-hydroxy ketones, a class to which 3-hydroxycyclopentanone belongs, are key motifs in the production of natural products of biological interest .
Mode of Action
It’s known that aldehydes and ketones can react with hydroxylamine to form oximes or hydrazine to form hydrazones . This suggests that 3-Hydroxycyclopentanone may interact with its targets through similar chemical reactions.
Biochemical Pathways
It’s known that cyclic β-hydroxy ketones are often found as valuable building blocks in the production of natural products of biological interest . This suggests that 3-Hydroxycyclopentanone may play a role in these biochemical pathways.
Result of Action
Given its potential role in the production of natural products of biological interest , it may contribute to various biological processes.
Action Environment
It’s known that the compound is stable under normal storage conditions (2-8°c) .
properties
IUPAC Name |
3-hydroxycyclopentan-1-one |
Source
|
---|---|---|
Details | Computed by LexiChem 2.6.6 (PubChem release 2019.06.18) | |
Source | PubChem | |
URL | https://pubchem.ncbi.nlm.nih.gov | |
Description | Data deposited in or computed by PubChem | |
InChI |
InChI=1S/C5H8O2/c6-4-1-2-5(7)3-4/h4,6H,1-3H2 |
Source
|
Details | Computed by InChI 1.0.5 (PubChem release 2019.06.18) | |
Source | PubChem | |
URL | https://pubchem.ncbi.nlm.nih.gov | |
Description | Data deposited in or computed by PubChem | |
InChI Key |
GULNLSGTYCQLLM-UHFFFAOYSA-N |
Source
|
Details | Computed by InChI 1.0.5 (PubChem release 2019.06.18) | |
Source | PubChem | |
URL | https://pubchem.ncbi.nlm.nih.gov | |
Description | Data deposited in or computed by PubChem | |
Canonical SMILES |
C1CC(=O)CC1O |
Source
|
Details | Computed by OEChem 2.1.5 (PubChem release 2019.06.18) | |
Source | PubChem | |
URL | https://pubchem.ncbi.nlm.nih.gov | |
Description | Data deposited in or computed by PubChem | |
Molecular Formula |
C5H8O2 |
Source
|
Details | Computed by PubChem 2.1 (PubChem release 2019.06.18) | |
Source | PubChem | |
URL | https://pubchem.ncbi.nlm.nih.gov | |
Description | Data deposited in or computed by PubChem | |
Molecular Weight |
100.12 g/mol |
Source
|
Details | Computed by PubChem 2.1 (PubChem release 2021.05.07) | |
Source | PubChem | |
URL | https://pubchem.ncbi.nlm.nih.gov | |
Description | Data deposited in or computed by PubChem | |
Product Name |
3-Hydroxycyclopentanone |
Q & A
Q1: What is the significance of 3-hydroxycyclopentanone in organic synthesis?
A1: 3-Hydroxycyclopentanone serves as a crucial building block for synthesizing diverse natural products and pharmaceuticals. Its structure, featuring both a hydroxyl and a ketone group, makes it highly versatile for further chemical transformations. For instance, it acts as a key intermediate in synthesizing prostaglandins, a group of lipid compounds with hormone-like effects .
Disclaimer and Information on In-Vitro Research Products
Please be aware that all articles and product information presented on BenchChem are intended solely for informational purposes. The products available for purchase on BenchChem are specifically designed for in-vitro studies, which are conducted outside of living organisms. In-vitro studies, derived from the Latin term "in glass," involve experiments performed in controlled laboratory settings using cells or tissues. It is important to note that these products are not categorized as medicines or drugs, and they have not received approval from the FDA for the prevention, treatment, or cure of any medical condition, ailment, or disease. We must emphasize that any form of bodily introduction of these products into humans or animals is strictly prohibited by law. It is essential to adhere to these guidelines to ensure compliance with legal and ethical standards in research and experimentation.