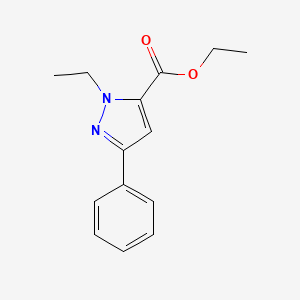
Ethyl 1-Ethyl-3-phenyl-1H-pyrazole-5-carboxylate
Overview
Description
Ethyl 1-Ethyl-3-phenyl-1H-pyrazole-5-carboxylate is a heterocyclic compound with the molecular formula C14H16N2O2. It is a member of the pyrazole family, which is known for its diverse applications in organic synthesis and medicinal chemistry . This compound is often used as a building block in the synthesis of more complex molecules due to its unique structural features.
Mechanism of Action
Target of Action
It is known that many bioactive aromatic compounds containing the indole nucleus, which is similar to the pyrazole nucleus in the compound, bind with high affinity to multiple receptors . These receptors play a crucial role in various biological processes.
Biochemical Pathways
It is known that indole derivatives, which are structurally similar to this compound, can affect a wide range of biological activities, including antiviral, anti-inflammatory, anticancer, anti-hiv, antioxidant, antimicrobial, antitubercular, antidiabetic, antimalarial, and anticholinesterase activities . The downstream effects of these activities would depend on the specific pathways involved.
Result of Action
Given the wide range of biological activities associated with similar compounds, it is likely that the effects would be diverse and could include changes in cell signaling, gene expression, and other cellular processes .
Action Environment
The action, efficacy, and stability of Ethyl 1-Ethyl-3-phenyl-1H-pyrazole-5-carboxylate could be influenced by various environmental factors . These could include the pH and temperature of the environment, the presence of other compounds, and specific characteristics of the target cells or organisms.
Biochemical Analysis
Biochemical Properties
Ethyl 1-Ethyl-3-phenyl-1H-pyrazole-5-carboxylate plays a significant role in biochemical reactions, particularly as an inhibitor of protein glycation. This compound interacts with various enzymes and proteins, including glycosyltransferases and oxidoreductases, which are crucial in metabolic pathways. The nature of these interactions often involves the inhibition of enzyme activity, leading to a decrease in the formation of advanced glycation end-products (AGEs). Additionally, this compound has been shown to exhibit antioxidant properties, further contributing to its biochemical significance .
Cellular Effects
This compound affects various types of cells and cellular processes. It has been observed to inhibit the proliferation of cancer cells, particularly in the A549 lung cancer cell line . This compound influences cell function by modulating cell signaling pathways, such as the mitogen-activated protein kinase (MAPK) pathway, and altering gene expression related to cell cycle regulation and apoptosis. Furthermore, this compound impacts cellular metabolism by reducing oxidative stress and enhancing the activity of antioxidant enzymes.
Molecular Mechanism
The molecular mechanism of this compound involves its interaction with specific biomolecules, leading to enzyme inhibition or activation. This compound binds to the active sites of enzymes, such as glycosyltransferases, through hydrogen bonding and hydrophobic interactions. This binding results in the inhibition of enzyme activity, thereby reducing the formation of AGEs. Additionally, this compound can modulate gene expression by interacting with transcription factors and influencing the transcriptional activity of genes involved in oxidative stress response .
Temporal Effects in Laboratory Settings
In laboratory settings, the effects of this compound have been observed to change over time. This compound exhibits good stability under standard laboratory conditions, with minimal degradation over extended periods. Long-term studies have shown that this compound maintains its inhibitory effects on enzyme activity and continues to reduce oxidative stress in cells. Prolonged exposure to this compound may lead to adaptive cellular responses, such as upregulation of antioxidant enzymes .
Dosage Effects in Animal Models
The effects of this compound vary with different dosages in animal models. At low doses, this compound exhibits beneficial effects, such as reducing oxidative stress and inhibiting cancer cell proliferation. At higher doses, this compound may induce toxic effects, including hepatotoxicity and nephrotoxicity. Threshold effects have been observed, where the compound’s beneficial effects plateau at a certain dosage, beyond which adverse effects become more prominent .
Metabolic Pathways
This compound is involved in several metabolic pathways, primarily those related to oxidative stress response and protein glycation. This compound interacts with enzymes such as glycosyltransferases and oxidoreductases, influencing metabolic flux and metabolite levels. By inhibiting glycosyltransferase activity, this compound reduces the formation of AGEs, thereby mitigating the adverse effects of protein glycation .
Transport and Distribution
Within cells and tissues, this compound is transported and distributed through passive diffusion and active transport mechanisms. This compound interacts with transporters and binding proteins, facilitating its localization to specific cellular compartments. The distribution of this compound is influenced by its lipophilicity, allowing it to accumulate in lipid-rich regions of cells and tissues .
Subcellular Localization
This compound exhibits specific subcellular localization, primarily targeting the cytoplasm and mitochondria. This localization is facilitated by targeting signals and post-translational modifications that direct the compound to these compartments. In the cytoplasm, this compound interacts with enzymes involved in oxidative stress response, while in the mitochondria, it modulates mitochondrial function and reduces oxidative damage .
Preparation Methods
Synthetic Routes and Reaction Conditions
The synthesis of Ethyl 1-Ethyl-3-phenyl-1H-pyrazole-5-carboxylate typically involves the condensation of 1,3-diketones with arylhydrazines . One common method includes the reaction of ethyl acetoacetate with phenylhydrazine under acidic conditions to form the pyrazole ring. The reaction is usually carried out in ethanol as a solvent, and the product is purified by recrystallization.
Industrial Production Methods
In an industrial setting, the production of this compound can be scaled up using continuous flow reactors. This method allows for better control over reaction conditions and higher yields. The use of catalysts such as nano-ZnO can further enhance the efficiency of the synthesis .
Chemical Reactions Analysis
Types of Reactions
Ethyl 1-Ethyl-3-phenyl-1H-pyrazole-5-carboxylate undergoes various chemical reactions, including:
Oxidation: The compound can be oxidized to form corresponding pyrazole oxides.
Reduction: Reduction reactions can yield hydropyrazoles.
Substitution: Electrophilic substitution reactions can introduce various functional groups onto the pyrazole ring.
Common Reagents and Conditions
Oxidation: Common oxidizing agents include potassium permanganate and hydrogen peroxide.
Reduction: Sodium borohydride and lithium aluminum hydride are frequently used reducing agents.
Substitution: Halogenating agents like bromine or chlorine can be used for electrophilic substitution reactions.
Major Products Formed
The major products formed from these reactions include substituted pyrazoles, pyrazole oxides, and hydropyrazoles, depending on the reaction conditions and reagents used .
Scientific Research Applications
Ethyl 1-Ethyl-3-phenyl-1H-pyrazole-5-carboxylate has a wide range of applications in scientific research:
Chemistry: It serves as a precursor for the synthesis of more complex heterocyclic compounds.
Biology: The compound is used in the study of enzyme inhibitors and receptor ligands.
Industry: The compound is used in the production of agrochemicals and dyes.
Comparison with Similar Compounds
Similar Compounds
- Methyl 1-ethyl-3-phenyl-1H-pyrazole-5-carboxylate
- Ethyl 5-chloro-1-phenyl-1H-pyrazole-4-carboxylate
- Ethyl 5-[3-(aminomethyl)phenyl]-1H-pyrazole-3-carboxylate hydrochloride
Uniqueness
This compound is unique due to its specific substitution pattern on the pyrazole ring, which imparts distinct chemical and biological properties. This uniqueness makes it a valuable compound in the synthesis of specialized molecules and in various research applications .
Properties
IUPAC Name |
ethyl 2-ethyl-5-phenylpyrazole-3-carboxylate | |
---|---|---|
Details | Computed by Lexichem TK 2.7.0 (PubChem release 2021.05.07) | |
Source | PubChem | |
URL | https://pubchem.ncbi.nlm.nih.gov | |
Description | Data deposited in or computed by PubChem | |
InChI |
InChI=1S/C14H16N2O2/c1-3-16-13(14(17)18-4-2)10-12(15-16)11-8-6-5-7-9-11/h5-10H,3-4H2,1-2H3 | |
Details | Computed by InChI 1.0.6 (PubChem release 2021.05.07) | |
Source | PubChem | |
URL | https://pubchem.ncbi.nlm.nih.gov | |
Description | Data deposited in or computed by PubChem | |
InChI Key |
PTGXNENSKKSZDH-UHFFFAOYSA-N | |
Details | Computed by InChI 1.0.6 (PubChem release 2021.05.07) | |
Source | PubChem | |
URL | https://pubchem.ncbi.nlm.nih.gov | |
Description | Data deposited in or computed by PubChem | |
Canonical SMILES |
CCN1C(=CC(=N1)C2=CC=CC=C2)C(=O)OCC | |
Details | Computed by OEChem 2.3.0 (PubChem release 2021.05.07) | |
Source | PubChem | |
URL | https://pubchem.ncbi.nlm.nih.gov | |
Description | Data deposited in or computed by PubChem | |
Molecular Formula |
C14H16N2O2 | |
Details | Computed by PubChem 2.1 (PubChem release 2021.05.07) | |
Source | PubChem | |
URL | https://pubchem.ncbi.nlm.nih.gov | |
Description | Data deposited in or computed by PubChem | |
Molecular Weight |
244.29 g/mol | |
Details | Computed by PubChem 2.1 (PubChem release 2021.05.07) | |
Source | PubChem | |
URL | https://pubchem.ncbi.nlm.nih.gov | |
Description | Data deposited in or computed by PubChem | |
Synthesis routes and methods
Procedure details
Disclaimer and Information on In-Vitro Research Products
Please be aware that all articles and product information presented on BenchChem are intended solely for informational purposes. The products available for purchase on BenchChem are specifically designed for in-vitro studies, which are conducted outside of living organisms. In-vitro studies, derived from the Latin term "in glass," involve experiments performed in controlled laboratory settings using cells or tissues. It is important to note that these products are not categorized as medicines or drugs, and they have not received approval from the FDA for the prevention, treatment, or cure of any medical condition, ailment, or disease. We must emphasize that any form of bodily introduction of these products into humans or animals is strictly prohibited by law. It is essential to adhere to these guidelines to ensure compliance with legal and ethical standards in research and experimentation.